Molecular Approaches to Taenia asiatica
Article information
Abstract
Taenia solium, T. saginata, and T. asiatica are taeniid tapeworms that cause taeniasis in humans and cysticercosis in intermediate host animals. Taeniases remain an important public health concerns in the world. Molecular diagnostic methods using PCR assays have been developed for rapid and accurate detection of human infecting taeniid tapeworms, including the use of sequence-specific DNA probes, PCR-RFLP, and multiplex PCR. More recently, DNA diagnosis using PCR based on histopathological specimens such as 10% formalin-fixed paraffin-embedded and stained sections mounted on slides has been applied to cestode infections. The mitochondrial gene sequence is believed to be a very useful molecular marker for not only studying evolutionary relationships among distantly related taxa, but also for investigating the phylo-biogeography of closely related species. The complete sequence of the human Taenia tapeworms mitochondrial genomes were determined, and its organization and structure were compared to other human-tropic Taenia tapeworms for which complete mitochondrial sequence data were available. The multiplex PCR assay with the Ta4978F, Ts5058F, Tso7421F, and Rev7915 primers will be useful for differential diagnosis, molecular characterization, and epidemiological surveys of human Taenia tapeworms.
INTRODUCTION
Taenia solium, T. saginata, and T. asiatica are taeniid tapeworms that cause taeniasis in humans and cause cysticercosis in intermediate host animals. T. asiatica is the last species found to be a human infecting tapeworm which has been reported in aspects of geographical distribution, epidemiology, and immunodiagnosis [1-3]. T. asiatica has been identified in Asian countries, i.e., China, Indonesia, Korea, the Philippines, Taiwan, Thailand, Vietnam, and Japan but this tapeworm has not been reported in Africa, Europe, and America. Analyses of phylogenetic relationships using morphologic characters and molecular data [4-6], the complete mitochondrial DNA (mtDNA) [7,8] indicated that T. asiatica is a distinct sister species to T. saginata.
Molecular approaches to differential diagnosis of human Taenia tapeworms have been developed, including the use of sequence-specific DNA probes [9-12], PCR-restriction fragment length polymorphisms (PCR-RFLP) [13-17], and multiplex PCR [18-20], nucleotide sequencing based on 10% formalin-fixed paraffin-embedded specimens [21,22], and DNA genotype [23,24].
The mitochondrial genome is one of the useful molecular marker resources for not only studying evolutionary relationships among distantly related taxa, but also for investigating the phylo-biogeography of closely related species, including species level taxonomy. The complete sequence of the human Taenia tapeworm mitochondrial genome was determined, and its complete sequence data, organization, and structure were available. The overall sequence difference in the full mitochondrial genome between T. asiatica and T. saginata was 4.6%, while T. solium differed by 11% [25]. The multiplex PCR assay with the Ta4978F, Ts5058F, Tso7421F, and Rev7915 primers using the full mitochondrial DNA sequence information were used for differential diagnosis, molecular characterization, and epidemiological surveys of T. asiatica, T. saginata, and T. solium [20].
The improvement of genomic DNA extraction methods from various tissues and copro-DNA sources is prerequisite for applying molecular genetic techniques to relevant study fields. The majority of Taenia tapeworm specimens were fixed in formalin fixatives in museum collections, laboratories, and institutes for permanent preservation. The eggs and particles of proglottids were released into the stool; molecular diagnosis of specimens preserved in formalin for a long time or eggs in stool can be useful in identifying the species even though the DNA molecules were degraded or very small in volume. High-resolution multiplex PCR assay and DNA sequencing based on formalin-fixed specimens or copro-DNA that could be used for differential diagnosis of human-infecting Taenia tapeworms.
GENETIC DIVERGENCE OF MITOCHONDRIAL GENOMES
The mitochondrial genomes of T. asiatica and T. saginata were each found to be approximately 14 kb in length and were amplified by long-PCR from 100 ng of total genomic DNA isolated from adult worms. The location of the oligonucleotide primers for cloning the complete mtDNA was used with universal forward primers from 69 to 129 bp, and reverse from 420 to 471 bp in the large subunit ribosomal RNA gene (16S rDNA) of T. solium. Variation in size of mtDNA restriction enzyme fragments data have become valuable tools for studying phylogenetic relationships at species levels [26]. Restriction enzyme cleavage maps of T. asiatica and T. saginata mitochondrial genomes were constructed by enzyme digests. The restriction fragments of the mitochondrial genome of T. asiatica digested with a variety of enzymes were as follows: 7, 5, 1.1, and 0.6 kb, AccI; 7, and 6.7 kb, AvaI; 10 and 4 kb, BamHI; 8, 4, and 2 kb, BglII; 5, 3.5, and 3 kb, EcoRV; 4.5, 3.8, 2.0, 1.6, and 1 kb, HincII; 9 and 5 kb, HindIII; 11 and 3 kb, KpnI; 9 and 5 kb, PstI; 9, 2.5, and 1.5 kb, PvuII; 9 and 5 kb, SalI. No enzyme sites for ClaI, EcoRI, KpnI, XbaI, and XhoI were observed in the T. asiatica mtDNA. The restriction enzyme map of T. saginata mtDNA was generated from the following restriction fragments: 8, 4, and 2kb, BglII; 4.5, 3.8, 1.5, and 1 kb, HincII; 5, 4.5, 2, and 1.5 kb, HindIII; 9 and 4 kb, PvuII; 12 and 1.8kb, XhoI. No enzyme sites for BamHI, EcoRI, EcoRV, KpnI, and PstI were observed in the T. saginata mtDNA. These restriction profiles were utilized to estimate the genetic divergence of 2 parasites, as the basis for the calculation of similarity indices and genetic variations, on the basis of percentage nucleotide sequence divergence. The genetic divergence of T. asiatica and T. saginata was observed by equations 20 and 21 [27]. The migration distances of the mtDNA fragments were observed from 46 fragments, including 12 fragments shared between T. asiatica and T. saginata. The full length of the mitochondrial sequence divergence between T. asiatica and T. saginata was estimated at 4.8% [8].
COMPLETE MITOCHONDRIAL GENOME OF HUMAN TAENIA TAPEWORMS
Gene content and organization
The complete mitochondrial sequence data of the human infecting Taenia tapeworms are available. The mitochondrial genome of human Taenia tapeworms was 13,703 bp (T. asiatica), 13,670 bp (T. saginata), and 13,709 bp (T. solium) long, containing 36 genes, of which 12 were protein-coding genes, 2 ribosomal RNA (rRNAs, a small and a large subunit), and 22 transfer RNAs (tRNAs). The mitochondrial genome lacked the atp8 gene. It was assumed that all genes were encoded in the direction and arranged the relative positions of gene loci in the mitochondrial genome of human Taenia tapeworms [25]. This mitochondrial genome was typical of those found in other platyhelminth mitochondrial genomes. The arrangement of mitochondrial genes in human Taenia tapeworms is identical to that of other cestodes, with the exception of Hymenolepis diminuta, in which trnL1 and trnS2 are reversed [28]. The proportion of the mitochondrial genome of human Taenia tapeworms consisting of protein-encoding genes is around 70%, and is similar to data for other cestodes. The nucleotide composition of the mitochondrial genome in human Taenia tapeworms is asymmetrical, with a positive GC-skew and a negative AT-skew. An A+T richness is observed in human Taenia tapeworms; T. asiatica is 72% A+T; T. saginata mtDNA is 71% A+T; and T. solium is 72% A+T (Table 1). Some genes overlap in the mitochondrial genomes of human Taenia tapeworms. A 34-bp region at the 3' end of T. asiatica and T. saginata nad4L overlapped the 5' end of nad4. Overlaps ranging from 10 bp (T. solium) to 15 bp (T. asiatica and T. saginata) have been observed in all 3 human Taenia tapeworms between the 3' end of cox1 and the 5' end of trnT. The non-coding regions of the mitochondrial genome of human Taenia tapeworms consisted of 2 regions; a short non-coding region (SNR) and a long non-coding region (LNR). These were located between trnY and trnL1(CUN), and nad5 and trnG, respectively.
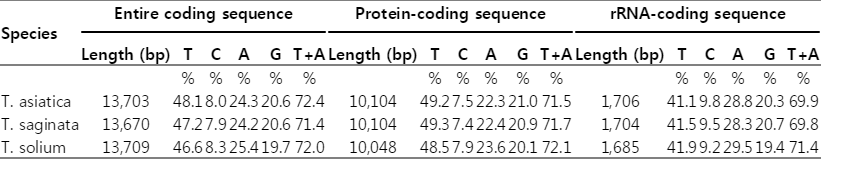
Nucleotide composition of the complete mitochondrial genome, protein-coding genes, and ribosomal RNA sequences of the human Taenia tapeworms
Human Taenia mtDNAs show an extreme bias towards T and against C, which results in a specific strand bias that influences the genetic code. The platyhelminth mitochondrial genetic code [29-31] was used to translate the mitochondrial protein-coding sequences. This code included the following modified genetic codon assignments: ATA=isoleucine; AG (A/G)=serine; TGA=tryptophan; and AAA=asparagine. Distinct differences have been inferred for platyhelminths, such as AAA encodes N rather than K, ATA encodes I rather than M, and TAA encodes Y rather than a termination [32]. Anticodon nucleotides are identical to those most commonly found in the amino acid of other mitochondrial genomes, with 2 exceptions. Lysine in the mitochondrial genomes of human Taenia tapeworms has a CTT (0.8-0.9%) anticodon rather than the more common TTT (11.6-11.8%), as is the case for each of the other flatworms whose mtDNA has been completely sequenced. A C in the wobble position would be consistent with the genetic code variation in which AAG specifies lysine. The GTT anticodon of asparagine in human Taenia tapeworm mitochondrial genomes would recognize both AAY and AAA. Arginine is specified by all 4 CGN codons and this N must pair with all 4 nucleotides (Table 2).
Nucleotide composition and codon usage
The nucleotide composition of human Taenia tapeworms comprised more than 70% AT of the total mitochondrial genome (Table 1). The predicted composition of amino acids encoded by the mitochondrial genome of human Taenia tapeworms was shown that phenylalanine (TTY), leucine (TTR, CTN), serine (AGN, TCN), and valine (GTN) constitute almost 50% of the total amino acid composition, while glutamine (CAR), lysine (AAG), histidine (CAY), and arginine (CGN) are the least abundant. This is due to the high T and low C content of the mtDNA, and the average frequent use of codons ending with T, A, G, and C (49%, 22.7%, 20.6%, and 7.6%, respectively) (Table 2). The bias towards the use of T and against the use of C is common in platyhelminths. Consistent with this pattern is the fact that phenylalanine, leucine, serine, and valine are common in the mitochondrial proteins of human Taenia tapeworms.
The mitochondrial genome of human Taenia tapeworms appears to use the flatworm mitochondrial code; namely, ATA for isoleucine, TGA for tryptophan, and AGR for serine [31]. All of the putative open reading frames (ORFs) of the 12 protein-coding genes of T. asiatica and T. saginata mtDNA started and ended with complete codons. The TAG stop codon was used in 4 genes (cox3, nad4L, nad4, and cox2), while the TAA termination codon was used in the remaining 8 genes (cob, atp6, nad1, nad2, nad3, nad5, nad6, and cox1). The use of a single T termination was found in nad1 for T. solium. A comparison was made of the protein-coding mitochondrial genes of all 3 human Taenia tapeworms [25]. An examination of initiation codon usage in T. saginata revealed that the ATG codon was used in 10 protein-coding genes, while the GTG codon was used in 2 genes (nad4 and atp6). The predicted number of amino acids encoded by each mitochondrial gene of human Taenia tapeworms does not vary, with the exception of nad1 (891 aa) and nad4 (1,212 aa) for T. solium, and cox2 (576 aa) for T. asiatica. Codon usage in the T. saginata mitochondrial genome is shown in Table 2. The 10 most commonly used codons are Phe (TTT), Leu (TTA), Ile (ATT and ATA), Tyr (TAT), Leu (TTG), Asn (ATT), Cys (TGT), Val (GTT), Ser (AGT), and Gly (GGT). They are all AT rich and 8 contain a thymidine in the third position. In contrast, the least used codons are Leu (CTT and CTC), Ile (ATC), Pro (CCC), Thr (ACC), Ala (GCC), His (CAC), Arg (CGT), Cys (TGC), and Gly (GGC), and generally contained a cystidine in the first or third position. Codons for Phe, Leu, Ser, and Val were the most common, with TTT being the most often used of the 4, making up 11.8% of all codons. The TCC codon for serine in T. saginata, the CTC codon for leucine in T. asiatica, and the CGC codon for arginine in T. solium were not used (Table 2).
All the ORFs of the 12 protein-coding genes of the T. asiatica and T. saginata mitochondrial genomes start and end by utilizing complete codons. However, 11 of the 12 ORFs of the mitochondrial genome in T. solium end with a complete stop codon (6 are TAG, and 5 are TAA), and 1 encoded by nad1 ends with an abbreviated T stop codon. In this case, a complete termination codon could not be generated, even with an overlap into the tRNA gene. This association between incomplete termination codons and the start of tRNA genes appears to be common in metazoan mtDNAs, where tRNAs of polycistronic transcripts serve as processing signals for cleavage into individual mRNAs [33,34]. In their analysis of the mitochondrial genome of H. diminuta, these authors inferred that cox1 terminated with an abbreviated stop codon T, and thus did not overlap the downstream trnT. They also pointed out that an in-frame stop codon (TAG) occurred downstream of the abbreviated codon, implying a 10 bp overlap with trnT if this were the true stop codon [28].
Transfer RNA and ribosomal RNA genes
A total of 22 tRNA genes were identified in the mitochondrial genome of human Taenia tapeworms. The length of the tRNA genes varied from 57 to 75 bp. The inferred secondary structure of 18 of the tRNAs shows the typical clover-leaf shape, with paired DHU arms. However, the predicted secondary structures of trnR, trnC, trnS1, and trnS2 have unpaired DHU arms, 7-16 bp in length. The amino-acyl acceptor arms in all of the predicted tRNA secondary structures of human Taenia tapeworms consisted of 7 bp. The acceptor arms of trnN, trnG, trnI, trnP, trnS1, trnS2, trnT, and trnV contained 1 or 2 non-canonical base pairs. The anticodon stems of 21 tRNAs were all 5 bp long, which is typical; however, the anticodon stem of trnN was 4 bp long, which could be due to a T : T mispair at the base of the stem in this tRNA. The anticodon loop consisted of 7 bp in all of the mitochondrial tRNAs of human Taenia tapeworms. The predicted secondary structures of the 22 tRNAs in T. asiatica and T. saginata have paired TΨC arms, consisting of a stem of 2-5 bp, with a 3-14 bp loop. The variable loop between the anticodon and the TΨC stems consists of 4 bases, except in cases involving trnR, trnC, trnS1, and trnS2. The most variable in length is trnS2(UGN), represented by 70 bp and 72 bp in T. asiatica and T. saginata, respectively, and 60 bp in T. solium [25]. The trnS tRNAs in other parasitic platyhelminths also lack a DHU arm, such as the trnS genes in a number of other metazoan mitochondrial genomes. These amino acids have C, A, and G in their wobble positions, as found in other flatworms.
The 2 mitochondrial ribosomal subunit genes, rrnL and rrnS, in the 3 human Taenia tapeworms are separated by trnC. The size was similar to those of rRNA genes in other human Taenia tapeworms, which range from 705 to 731 bp for rrnS, and 972 to 980 bp for rrnL (Table 1). The average of nucleotide content of the rrn genes in human Taenia tapeworms was 28.8% A, 41.5% T, 20.1% G, 9.5% C, and 70.3% A+T.
Non-coding regions
The mtDNA of human Taenia tapeworms contained 2 major non-coding regions. The short non-coding region between trnY(UGN) and trnL1(UUN) varied from 66 bp to 71 bp in length, and had more than 70% A+T content. This region consisted of 2 putative hairpin structures, which can fold into a stem-and-loop structure. The long non-coding region between nad5 and trnG varied from 159 bp to 192 bp in length, and had more than 75% A+T content. Apart from short intergenic sequences ranging from 1 to 25 bp, they are located between trnS2(UGN) and trnL2(UUN) in T. saginata, trnL2(UUN) and trnR in T. asiatica, and nad2 and trnV in T. solium. There are 2 other intergenic or non-coding regions (NR) that are functionally unassigned. The non-coding regions in these 3 species varied in size from 66 to 71 bp in the SNR, and from 159 to 192 bp in the LNR. This region contained 4 putative hairpin structures with conserved sequences, TATA and GAA(A)T [25]. The stem-and-loop structure in this region suggests that it may be involved in regulating light-strand replication origins in vertebrate mtDNAs [22].
Mitochondrial sequence divergence among the human Taenia tapeworms
The divergences of 3 human Taenia tapeworm mtDNAs were estimated by the percentage of nucleotide sequence differences and predicted amino acids [25]. The protein-coding sequences of T. asiatica and T. saginata contained 10,104 bps and 3,368 codons, while those of T. solium contain 10,048 bps and 3,349 codons, respectively. The 12 protein-coding genes of T. asiatica and T. saginata differed by 4.6%, while the overall difference between T. asiatica and T. saginata across the entire mtDNA sequence was 4.6%. The percentage pairwise divergences of nucleotides among the human Taenia tapeworms ranged from a low of 3.0% to a high of 27.9%. Among the protein-coding genes of T. asiatica and T. saginata that showed an average pairwise similarity of about 95%, nad5 was the most variable gene. A higher degree of conservation was found in genes encoding the 3 subunits of cytochrome c oxidase, cytochrome b, 16S rRNA, and the tRNAs. Both of the rrn genes of T. asiatica differed by 5% and 12% from T. saginata and T. solium, respectively. The percent nucleotide difference was more than the percent amino acid divergence for cox1, cox2, cox3, cob, nad1, nad4L, and nad5 genes, implying the existence of non-synonymous substitutions. Two classes of protein-coding genes can be identified in the mitochondrial genome of the human Taenia tapeworms; a slow-evolving gene of non-synonymous substitution sites that includes tRNAs, rRNAs, and D-loop domains, and a fast-evolving gene of synonymous substitution sites that includes atp6 and nad6.
The sequence divergence of the mitochondrial genomes between T. asiatica and T. saginata was estimated to be 4.8% by restriction enzyme analysis [8]. A pairwise comparison of nucleotide sequences was conducted with the mitochondrial genomes of the 3 human Taenia tapeworms. The overall sequence difference between T. asiatica and T. saginata was 4.6%, while that between T. saginata and T. solium was 11%. The degree of divergence in mtDNA sequence was estimated using the genetic distance of the cob gene between sister species, congeneric species and confamilial genera. There is more than a 2% sequence divergence among amphibian, reptile, avian, and mammalian groups [35]. Closely related species of vertebrates regularly show more than 2% divergence for another mitochondrial gene, cytochrome b, while intraspecific divergences are rarely greater than 2%, and most are less than 1% [36].
DIFFERENTIAL DIAGNOSIS OF HUMAN TAENIA TAPEWORMS USING MULTIPLEX PCR
Early detection and adequate treatment of taeniasis is important not only for the prevention of cysticercosis, but also for generating reliable epidemiologic information and effective control of taeniasis and cysticercosis. Due to their morphological similarities and sympatric distribution patterns in Asian regions, there is frequent confusion between T. saginata and T. asiatica. More recently, molecular approaches to differential diagnosis of T. saginata and T. asiatica have been developed, including the use of sequence-specific DNA probes, PCR coupled to RFLP [13,16,17], or multiplex PCR [19,20]. Until recent introduction of PCR or multiplex PCR assays, species-specific diagnosis of human taeniasis (T. solium, T. saginata, and T. asiatica) was problematic. Therefore, a high-resolution multiplex PCR assay was developed for differential diagnosis of human-infecting Taenia tapeworms.
Three species-specific forward primers were prepared based on nucleotide sequences of valine transfer RNA and NADH dehydrogenase subunit 2 from human taeniid cestodes. The 3 forward primers were designed to amplify different-sized products; Ta7216F, specific for T. asiatica (5'-GGG TTT AAG TTA TAA ATG TGA TGT-3', nucleotides 4,995 to 5,016 from GenBank accession no. AF445798); Ts7313F, specific for T. saginata (5'-GGG TTT AAG TTA TAA ATG TGA TGT-3', nucleotides 5,057 to 5,080 from AY684274); and Tso7466F, specific for T. solium (5'-CTA GGC CAC TTA GTA GTT TAG TTA-3', nucleotides 7,431 to 7,456 from AB086256). The reverse primer, Rev7915, (5'-CAT AAA ACA CTC AAA CCT TAT AGA-3', nucleotides 7,869 to 7,893 from AF445798, AY684274, AB086256), was from a highly conserved sequence common to all of these cestodes. Species-specific product with molecular sizes of 706 bp, 629 bp, and 474 bp were amplified from T. asiatica, T. saginata, and T. solium in regular PCR reaction, respectively. In order to test differential diagnosis of Taenia tapeworm infections at one time, multiplex PCR was performed using mixture of all primers and genomic DNA of Taenia tapeworms. Three set of primers (Ta7216F, Ts7313F, Tso7466F, and Rev7915) were combined in a single reaction using a mixture of template DNA of 3 Taenia tapeworms. When multiplex PCR was performed with a mixture of T. asiatica, T. saginata, and T. solium, template DNA using the species-specific primer sets, PCR products of different size generated species-specific bands. The diagnostic, species-specific, PCR fragments derived using species-specific primers were similar to those obtained using multiplex PCR. This differential diagnosis by multiplex PCR provided better resolution of human Taenia tapeworms. PCR products derived from DNA isolated from fecal samples were detectable using a minimum concentration of 10 eggs per 1 g of human Taenia infections, and target gene fragments could be reliably detected at a concentration of 10 eggs or more [20].
MOLECULAR EPIDEMIOLOGIC STUDY OF HUMAN TAENIA TAPEWORMS
Only 2 types of taeniases (T. saginata and T. solium) were recognized morphologically in Korea up to 1992 until T. asiatica being newly described in 1993 as the third type. The prevalence of taeniasis in Korea was considered to be caused only by infection with T. saginata or T. solium until Eom and Rim revised the identification of the Korean Taenia tapeworms to establish a new species, T. asiatica [1]. In order to understand distribution of human Taenia tapeworms in Korea, 68 Taenia specimens were analyzed by morphology and nucleotide sequences of mitochondrial cox1 gene and ITS2 region. The distribution ratio of T. solium : T. asiatica : T. saginata was 3:51:14 (or approximately 1:18:5) from 9 provinces in Korea between 1935 and 2005 [37].
Regarding taeniases in other Asian countries, intestinal parasite control among primary school children have been performed in Cambodia by Korea Association of Health Promotion and National Center for Parasitology, Entomology, and Malaria Control since 2006. Taenia tapeworm eggs were collected from 21 Taenia tapeworm carriers in Koh Kong inhabitants [23]. The Taenia eggs were isolated from the stools and placed in DNA sequencing and multiplex PCR for differentiated diagnosis. All of the 21 Taenia specimens from Koh Kong in Cambodia were identified as T. saginata (n=19) and T. solium (n=2) by cox1 sequencing and multiplex PCR.
The international collaboration project between Korea and Lao People's Democratic Republic (Lao PDR) was performed intestinal parasite control among primary schoolchildren (2000-2004) by Korea Association of Health Promotion (KAHP) and Korea International Cooperation Agency (KOICA), Korea-Lao PDR collaborative project for control of food-borne trematode infections in Lao PDR (2007-2012) by Korea Foundation for International Healthcare (KFIH), KOICA, and Ministry of Health, Lao PDR. The status of human taeniasis in Lao PDR has been studied in Vientiane, Khammouane, and Champasak Province from 1989 to 2002 [38-41]. A nationwide survey was conducted in 2000-2002 using the cello-tape anal swab and the Kato-Katz fecal examination [42]. A total of 814 persons from 13 villages in Saravane Province in 2004 were investigated, and the overall prevalence of taeniasis was observed to be 5% [43]. These data were confirmed by coprological examination of eggs or expelled proglottids. However, there had been no information on differential identification of Taenia species in Lao PDR. Molecular identification of Taenia tapeworms was performed by multiplex PCR and DNA sequences that correspond to position 90-530 of cox1 gene. T. solium was detected in 3 samples and T. saginata in the remaining 117 samples (to be published). T. solium and T. saginata were recognized, and the disease complex of T. solium taeniasis/cysticercosis has been recognized to be present in Lao PDR.
The majority of Taenia tapeworm specimens in the museum collections are fixed in formalin fixatives for permanent preservation and morphological examinations. Formalin treatment of the specimen is generally known to lower the yields of genomic DNA due to its acceleration of the cross-linking between protein and DNA, posing an imperfection to use the DNA contents for a variety of scientific purposes, including molecular studies. Ten Taenia specimens were collected from Natural History Museum, Switzerland, and 3 specimens were donated from Dr. Yosuke Yamane in Japan. All specimens were preserved in 10% formalin between the years 1929 and 1996. PCR amplification and direct sequencing for the cox1 target fragment (349 bp in length corresponding to the positions 80-428 bp of the cox1 gene) were performed using the total genomic DNA extracted from formalin-preserved samples. PCR amplification was very successful in most case and generated high quality PCR product applicable to direct sequencing. Direct sequencing of the target fragment made it possible to molecularly discriminate 3 Taenia species. T. solium and T. saginata were detected from China and Swiss samples, and T. asiatica from Indonesia, Korea, and Japan [22].
A Korean non-governmental organization (NGO), Good Neighbors International, funded a survey in Tanzania for neglected tropical diseases, including helminth infections. Between 2005 and 2009, South Korea and Tanzania conducted a collaborative project to promote health through control of parasites among school children. In 2008 and 2009, we conducted a field investigation for helminth infections by examining stools and performing dot-ELISA screening for T. solium. Helminths and cestode infection cases were found by stool examination, Taenia-specific coproantigen tests, morphological observation, nucleotide analyses for sequence variations, and multiplex PCR for identification of human Taenia tapeworms. Five Taenia cases were found in Kongwa of Dodoma region and Mbulu districts in Arusha region, Tanzania. T. solium (n=1) and T. saginata (n=4) specimens were identified by mitochondrial cox1 sequencing and multiplex PCR [24].