Efficacy of a DNA Vaccine Carrying Eimeria maxima Gam56 Antigen Gene against Coccidiosis in Chickens
Article information
Abstract
To control coccidiosis without using prophylactic medications, a DNA vaccine targeting the gametophyte antigen Gam56 from Eimeria maxima in chickens was constructed, and the immunogenicity and protective effects were evaluated. The ORF of Gam56 gene was cloned into an eukaryotic expression vector pcDNA3.1(zeo)+. Expression of Gam56 protein in COS-7 cells transfected with recombinant plasmid pcDNA-Gam56 was confirmed by indirect immunofluorescence assay. The DNA vaccine was injected intramuscularly to yellow feathered broilers of 1-week old at 3 dosages (25, 50, and 100 µg/chick). Injection was repeated once 1 week later. One week after the second injection, birds were challenged orally with 5×104 sporulated oocysts of E. maxima, then weighed and killed at day 8 post challenge. Blood samples were collected and examined for specific peripheral blood lymphocyte proliferation activity and serum antibody levels. Compared with control groups, the administration of pcDNA-Gam56 vaccine markedly increased the lymphocyte proliferation activity (P<0.05) at day 7 and 14 after the first immunization. The level of lymphocyte proliferation started to decrease on day 21 after the first immunization. A similar trend was seen in specific antibody levels. Among the 3 pcDNA-Gam56 immunized groups, the median dosage group displayed the highest lymphocyte proliferation and antibody levels (P<0.05). The median dosage group had the greatest relative body weight gain (89.7%), and the greatest oocyst shedding reduction (53.7%). These results indicate that median dosage of DNA vaccine had good immunogenicity and immune protection effects, and may be used in field applications for coccidiosis control.
INTRODUCTION
Coccidiosis is a serious intestinal disease in chickens caused by 7 species of Eimeria protozoan parasites. It has been estimated that annual loss due to coccidiosis exceeds 2 billion US dollars worldwide [1]. Currently, coccidiosis is prevented and treated mainly with drugs. However, due to wide use of the drugs, drug resistance and drug residue are serious problems. These issues have led to the requirement for an effective vaccine strategy [2,3].
Live vaccines are the predominant vaccines for coccidiosis control. Although they have been used for many years, their application is limited due to the potential pathogenicity, high cost, laborious immunization procedure and demanding operational and management requirements [4,5]. Subunit vaccines and DNA vaccines may be good alternatives [3].
DNA vaccine is the third generation vaccine. Compared with the traditional ones, DNA vaccines can elicit immune responses fully and persistently without potential to cause the disease. They are simple and less laborious to prepare and transport. DNA vaccines have been called third revolution in vaccinology history, afford the focus of vaccine research. It has been applied in poultry infectious diseases and cancerous diseases, and positive effects were reported [6-8]. The application of DNA vaccines in chicken coccidiosis prevention has been reported. The targeted genes include MZ5-7 and SO7 of Eimeria tenella [9,10], lactate dehydrogenase, cSZ-2 and 3-1E gene of Eimeria acervulina [11-13], and the advances are encouraging.
Gam56 is an antigen produced during gametophyte stage of Eimeria maxima. It is one of the several confirmed proteins involved in oocyst wall formation, contains a tyrosine-rich region [14-16]. Similar regions were also found in its homologs in E. tenella and E. acervulina [17,18]. Gam56 has good antigenicity and immunogenicity [19]. It has been used as one of the main components in the subunit vaccine Cox Abic® for chicken coccidiosis, which contains 3 antigens with molecular respective weights 230, 82, and 56 kDa. These antigens are isolated from gametophyte of E. maxima using affinity chromatography. Immunization with this vaccine can reduce oocyst production by 50-80% in clinic applications [20]. Because these antigens are purified from intestinal epithelial cells of infected chickens, the production of this vaccine is complicated, time-consuming and expensive, which limits its application. Recently, Gam56 antigen was expressed in E. coli, the expression product has certain protective effects in chickens challenged with E. maxima. It could improve body weight gain, reduce intestinal lesions, and repress oocyst shedding by 57.8% [21]. These results indicated that Gam56 is a good candidate for genetically engineered vaccine against coccidiosis.
In this study, a DNA vaccine expressing Gam56 was constructed, and its immunogenicity and protective effects in chickens were evaluated. This study is of interest for clinical applications of DNA vaccine against coccidiosis.
MATERIALS AND METHODS
Parasite, animals, plasmids, and antisera
Yellow feathered broilers at 1-day of age were obtained from the Poultry Institute of China Agricultural Academy (Yangzhou, Jiangsu, China). The duration of study was 28 days. During the period of experiment, chicks were housed in rigorously decontaminated cages under coccidia-free conditions, provided with complete feed and clean water without anticoccidial drugs. Animal care and all experiments were performed according to the rules of the Animal Experiment Ethics Committee of Yangzhou University. Wild type E. maxima NT strain was isolated from Nantong of Jiangsu province, preserved in the Key Laboratory for Avian Preventive Medicine at Yangzhou University. Parasites were propagated in chickens, purified and counted with the conventional method [22].
Plasmid pGEM-T-Gam56 containing Gam56 cDNA was constructed in a previous study [21]. Mouse-anti-Gam56 serum was prepared as follows: Gam56 protein was expressed by a recombinant vector pGEX-6P-1 containing the Gam56 gene in E. coli and purified by affinity chromatography. Then, the Gam56 antigen was mixed with Freund's complete adjuvant, injected intramuscularly (i. m.) to ICR mice at 6 weeks of age. The injection was repeated at 1 week after the first injection and then serum was separated at 1 week after the second injection, which was tested and frozen in aliquots [21].
Construction of DNA vaccine plasmid
ORF of Gam56 cDNA was amplified with a pair of primers, P1 5'-CCCAAGCTTACCATGGCCCGCCTCGGCCTCG-3' (italicized was the HindIII site) and P2 5'-CCCGAATTCGGTCGTTTAGAAGGGCATGG-3' (italicized was the EcoRI site). A Kozark sequence was introduced at the region of ATG start codon. Plasmid pGEM-T-Gam56 was used as the PCR template. Thermal cycles of the PCR were: 5 min at 95℃; 30 cycles of denaturation (94℃ for 1 min), annealing (60℃ for 1 min), extension (72℃ for 2 min); a final extension at 72℃ for 10 min; and held at 4℃. The PCR product was purified after 1% agarose gel electrophoresis with a QIAquick kit (Qiagen, Hilden, Germany), digested with EcoRI and HindIII, ligated to eukaryotic expression vector pcDNA3.1(zeo)+ (Invitrogen Life Technologies, Carlsbad, California, USA) that was also digested with the 2 enzymes. DH5α was used as the host cell. The recombinant plasmid was assessed with EcoRI and HindIII digestion. The positive plasmid was designated pcDNA-Gam56, prepared with EndoFree Plasmid Giga Kit in large-scale (Qiagen).
Expression of DNA vaccine in vitro
COS-7 cells were transfected with pcDNA-Gam56 using lipofectin (Gibco BRL, Rockville, Maryland, USA). Briefly, a mixture of 100 µl of serum-free DMEM media (Gibco BRL) with 4 µg positive recombinant plasmid or 10 µl lipofectin was prepared in A and B tubes, set for 45 min at room temperature. Then, the 2 tubes were mixed and set again for 15 min. The mixture was then overlaid onto COS-7 cells that were grown in 35 mm plate and washed twice with serum-free DMEM. After 6 hr of transfection, cells were transferred to DMEM containing 10% calf serum, and cultured for 72 hr at 37℃ in 5% CO2.
Gam56 expression was assessed with indirect immunofluorescence assay. Briefly, transfected COS-7 cells were fixed in acetone-ethanol (1:1) fixative for 5 min, washed twice with PBS (0.05M, pH 7.4). After air drying, cells were incubated with 1:50 diluted mouse-anti-Gam56 serum at 37℃ for 45 min, washed 3 times with PBS, then incubated with 1:100 diluted, fluoroscein isothiocyanate (FITC)-labeled goat-anti-mouse IgG (Sigma; St. Louis, Missouri, USA) at 37℃ for 45 min. Subsequently, cells were washed 3 times with PBS, examined under fluorescence microscope and photographed. A control experiment was done with the empty vector pcDNA3.1.
DNA vaccination and challenge
Chicks were weighed at 7 days of age, assigned at random to 6 groups each with 40 chickens, 20 for immunization and challenge, 20 for immunization and detection of lymphocyte proliferation and antibody responses. Group 1 was neither immunized nor challenged, served as unchallenged control. Group 2 was unimmunized, served as challenged control. Group 3 was treated with pcDNA3.1 plasmid, served as plasmid control. Groups 4, 5, and 6 were treated with pcDNA-Gam56 at 25 µg (low dose), 50 µg (median dose), and 100 µg (high dose) per chick, respectively. Chicks were first injected via chest muscle with 0.2 ml of 25% sucrose, massaged 10 min, and then injected with plasmid at the same spot. Injection was repeated at 14 and 21 days of age. At 21 days of age, a half (20) of the birds except those in unimmunized and unchallenged group were challenged with 5×104 E. maxima NT oocyst, and those used for detection of lymphocyte proliferation and antibody responses were not challenged. Clinical signs and mortality of each group were observed and documented daily post challenge. Feces of each group were collected separately at days 5-8 post-infection. Chickens in all groups were weighed and euthanized on day 8 post challenge. The details for grouping and experimental design were shown in Table 1.
Lymphocyte proliferation assay
Peripheral blood was collected randomly from 5 chickens per group by cardiac puncture after euthanasia at 7, 14, 21, and 28 days of age. Peripheral blood lymphocytes were isolated with lymphocyte separation medium and adjusted to 1.0×107 cells/ml in RPMI-1640 medium (Gibco) containing 10% fetal calf serum, 100 U/ml penicillin, and 100 mg/ml streptomycin.
For lymphocyte proliferation assay, 96-well flat-bottomed plates (Costar, Cambridge, Massachusetts, USA) were used. Cells prepared as above were loaded (100 µl/well) to culture plates, cultured at 37℃ in 5% CO2 with 20 ml Gam56 protein (final concentration: 10 mg/ml) for 56 hr. MTT 5 mg/ml (AMRESCO, Solon, Ohio, USA) solution was added to the plate (10 µl per well) and incubated for 4 hr. The reaction was stopped by the addition of 100 ml dimethyl sulfoxide (DMSO), and the optical density was measured in an ELISA reader (Tecan, Seestrasse, Maennedorf, Switzerland) at 570 nm (OD570). Each sample was analyzed in triplicate.
Serum antibody response
Peripheral blood was collected as described in the lymphocyte proliferation assay. Serum was separated by low speed centrifugation, and ELISA was used to measure the Gam56-specific antibody responses. In brief, 96-well microtiter plates were coated overnight with 1 µg/well of purified recombinant Gam56 protein in carbonate buffer (0.05 M, pH 9.6). The plates were blocked with PBS buffer containing 10% fetal calf serum for 1 hr at 37℃. Serum sample was diluted 1:200, loaded to microtiter plate (100 µl/well), and incubated for 1.5 hr at 37℃. After washing 3 times with PBS containing 0.05% Tween, peroxidase-conjugated goat anti-chicken IgG (Sigma) diluted to 1:10,000 was added (100 µl/well) and incubated for 1 hr at 37℃. After washing, orthophenylene diamine (Sigma) (0.4 mg/ml in 0.05 M phosphate citrate buffer, pH 5.0) was added (100 µl/well) and incubated for 15 min at 37℃ to detect peroxidase activity. Finally, the reaction was stopped by the addition of 50 µl of 2 M sulfuric acid. Optical density at 490 nm (OD490) was measured with an automated microplate reader. All samples were analyzed in triplicate.
Evaluation of immune protection
Efficacy of immunization was evaluated on the basis of the survival rate, body weight gain (BWG), and oocyst shedding. The survival rate was estimated by the number of surviving chickens divided by the number of initial chickens. BWG was determined at the beginning of the challenge, and at the end of the challenge. The relative weight gain (%) was calculated as follows: 100× (the average body weight gain from vaccinated group/the average body weight gain from unchallenged control group). The total oocyst number in feces of each group was counted using the McMaster method modified by Talebi and Mulcahy [23], and each sample was counted 3 times. The reduction (%) in oocyst shedding was calculated as follows: (the average number of oocysts from challenged control group-the average number of oocysts from vaccinated group)/the average number of oocysts from challenged control group ×100.
Statistical analysis
Data were analyzed by SPSS software (SPSS 15.0 K for Windows, Chicago, Illinois, USA). All data were expressed as the mean±SD values. Differences between the groups were determined using ANOVA. The Duncan's multiple range test was used to analyze differences between the mean values, and differences were considered statistically significant at P<0.05.
RESULTS
Construction of plasmid pcDNA-Gam56
By using plasmid pGEM-T-Gam56 that contains a cDNA fragment from E. maxima as the template, with 2 primers designed, the Gam56 ORF was succefully amplified, and the expected 1,465 bp segment was obtained. This segment was cloned in an expression vector pcDNA3.1. The recombinant plasmid was assessed with EcoRI and HindIII double digestion. Results of sequence analysis indicated that the insert was exactly the same as that found in E. maxima Houghton strain (GenBank no. AY129951).
Expression of Gam56 protein in COS-7 cells
The expression of Gam56 antigen in COS-7 cells was examined with indirect fluorescent antibody (IFA) technique. Results indicated that Gam56 protein was expressed in COS-7 cells, with signals from the cytoplasm and membrane, displaying spreading fluorescence (Fig. 1A). Cells transfected with pcDNA3.1 displayed a negative signal (Fig. 1B).
Lymphocyte proliferation
The cell-mediated proliferation patterns to Gam56 protein as indicated by MTT in chickens are described in Fig. 2. Compared with the pcDNA control or non-immunized control, birds treated with pcDNA-Gam56 at each of the 3 dosages showed markedly increased (P<0.05) lymphocyte proliferation activity in peripheral blood lymphocytes at day 7 post-immunization (PI). Among the 3 dosage groups, the median group (50 µg) showed the highest (P<0.05) proliferation activity. The lymphocyte proliferation activity was maintained on day 14 PI, with the median group showing the highest value also (P<0.05). On day 21PI, the lymphocyte proliferation activity of peripheral blood lymphocytes in the vaccineated birds started to decrease but it still higher than that of the 3 control groups.
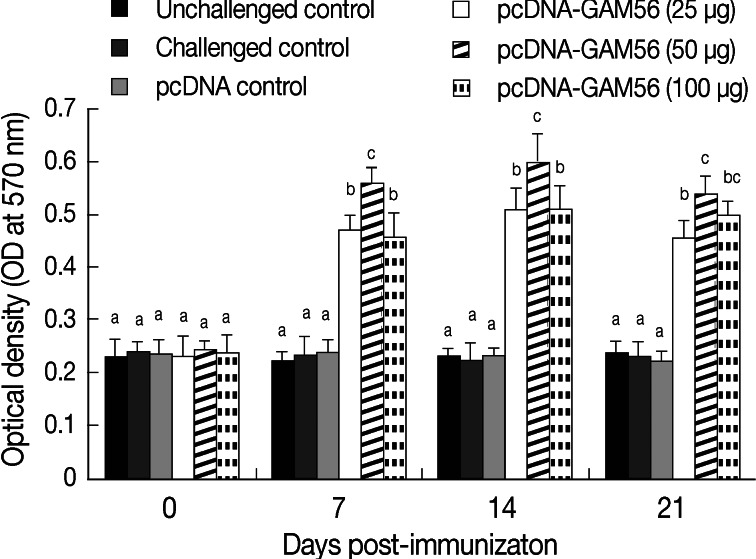
Lymphocyte proliferation activity in response to the DNA vaccination. The mean OD570 values of stimulated lymphocytes measured by MTT (n=5). Result was detected at 570 nm using an ELISA reader. Bars not sharing the indicated letters (a, b, and c) at the same time point are significantly different (P<0.05).
Serum antibody response
Serum antibody levels after DNA vaccine treatment were shown in Fig. 3. Compared with the pcDNA control or non-immunized control, birds treated with each of the 3 dosages of pcDNA-Gam56 plasmid showed markedly increased antibody levels (P<0.05) on day 7 PI. Among the 3 dosage groups, the median group showed the highest antibody level (P<0.05). Antibody levels kept increasing on day 14 PI, with the median group still being the highest (P<0.05). On day 21 PI, antibody levels started to decrease but were still higher than that of the 3 control groups.
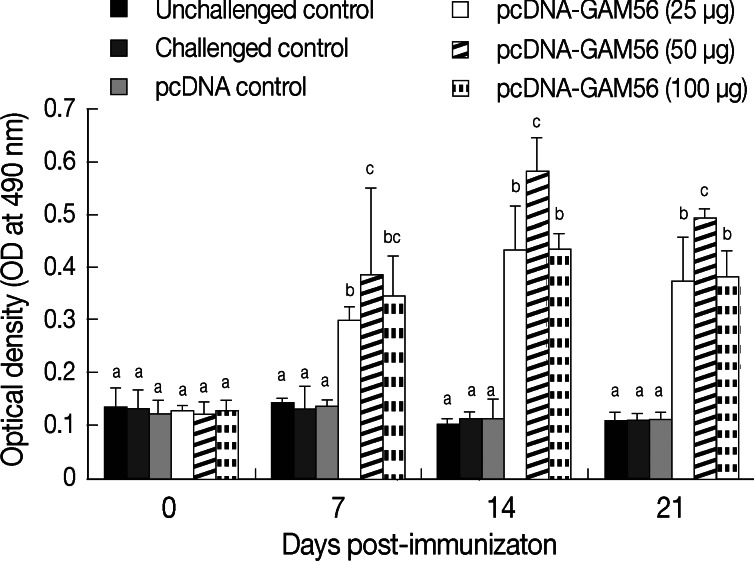
Antibody responses in chickens immunized with the DNA vaccine. Serum sample was diluted 1:200, and antibody was measured by indirect ELISA (n=5). Results were detected at 490 nm using an ELISA reader. Bars not sharing the indicated letters (a, b, and c) at the same time point are significantly different (P<0.05).
Protective effects of DNA vaccination against E. maxima challenge
On day 4 after E. maxima challenge, chickens started to display reduced appetite, reduced water intake, ruffled up feathers, reduced movement, apathetic, and diarrhea. No death occurred in all challenged groups. On day 8 post challenge, these symptoms disappeared. The challenged control group showed the highest number of diarrheal discharges, while the group with the median dosage immunization showed the least. The other 2 immunized groups showed slightly (insignificant) higher numbers of diarrheal discharges. The unchallenged control group displayed normal behavior during the entire period.
The immunizing efficacy of DNA vaccine in terms of BWG and oocyst output was shown in Table 2. Compared with the controls that were challenged without immunization or with pcDNA injection, groups treated with pcDNA-Gam56 showed higher BWG (P<0.05). Among the 3 immunized groups, the low dosage group showed the lowest BWG (P<0.05). The median dosage group showed the highest BWG with relative body weight gain reached to 89.7%, but were insignificant compared with the high dosage group (P>0.05).
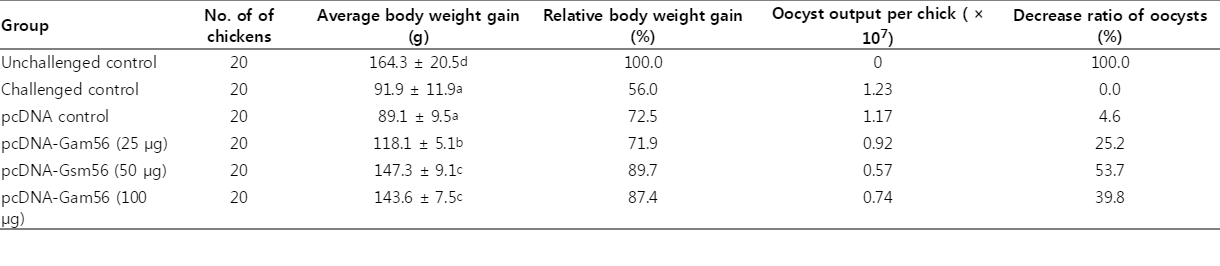
Protective effects of the constructed DNA vaccine sagainst experimental infection of Eimeria maxima in chickens
All challenged groups shed oocysts at various levels. There was only 4.6% reduction in the number of oocysts in the group treated with pcDNA3.1 plasmids. Among groups immunized with pcDNA-Gam56, the highest reduction was seen in chickens immunized with middle dose of DNA vaccine, which reched to 53.7%. Other 2 goups (25 µg and 100 µg) had reduction of 25.2% and 39.8%, respectively.
DISCUSSION
DNA vaccines have successfully induced protective immunity against various pathogens in many species [8]. In this study, a DNA vaccine targeting Gam56 has been engineered. The coding region of Gam56 gene of E. maxima was cloned in pcDNA3.1(zeo)+ vector under the control of CMV late enhancer promoter. In order to increase the expression efficiency and protective efficiency of the DNA vaccine, a Kozak sequence was introduced to the plasmid near the ATG start codon. Before in vivo experiments to verify the immunogenicity as well as the protective efficacy of DNA vaccine, a transfection experiment in vitro was conducted to assess the expression of engineered Gam56. Results indicated that the DNA vaccine was correctly constructed and expressed. The experiment in vivo further demonstrated that the DNA vaccine could elicit specific cellular and humoral immune responses, to some extent prevented chicken weight loss after E. maxima challenge and reduced oocyst discharge. These results further indicated that Gam56 antigen is an effective vaccine candidate. The immunity of DNA vaccines against Eimeria is species-specific [1]. Cross protection against other species of chicken coccidia is clearly needed.
Antigens from asexual reproduction stage have stronger immunogenicity than those from sexual reproduction stage. Target genes for vaccine development were often from asexual stage, or involved in invasion. Very few genes for vaccine development were antigens from sexual reproduction stage [5]. As an antigen from gametophyte stage, Gam56 plays a very important role in oocyst wall formation [18]. If the process of oocyst wall formation could be suppressed by immunization, it would be possible to block the life cycle of Eimeria and reduce oocyst discharge. Results of this study confirmed this postulation. Other proteins (Gam82 and Gam 230) also participate in the development of the oocyst wall [20]. Both Gam82 and Gam56 proteins are main target antigens that could induce transmission-blocking immunity [21,24]. DNA vaccine with multiple epitopes from these proteins could further improve the immune efficacy.
Infection with coccidial parasites elicits humoral and cell-mediated immune responses, although the relative roles of each in conferring resistance to coccidiosis are debatable. Nevertheless, it is clear that cellular responses are mediated by production of proinflammatory factors, Th1- and Th2-type cytokines [25]. Among their diverse functions, cytokines are potent adjuvants that amplify protective immunity to coccidial parasites. Compared with chicken embros vaccinated with recombinant EtMIC2 protein alone, significantly decreased oocyst shedding and improved weight gains were observed in those vaccinated with recombinant EtMIC2 protein pulsed with IL-8, IL-16, or TGF-β [26]. In the application of cytokines as adjuvants, their rapid degradation and clearance might decrease their activities [27,28]. Recently, co-expressing antigens of coccidial parasites and cytokines in DNA vaccines have been successfully achieved, which could overcome this problem and provide a more practical method for treating the large trail flock [9-13].
It was reported that the route, dosage, age, and time of administration affect the efficacy of the DNA vaccine. Optimization of vaccination protocols is expected to improve the efficacy [29]. Among these factors, under certain delivery methods, dosage is most important. It is generally believed that more plasmid could elicit stronger response. When chickens vaccinated with 12.5, 25, and 50 µg pcDNA3.1-Et1A-TA4 intramuscularly, the highest ACI (should be fully spelled) was obtained in 50 µg group [30]. However, this linear dose relationship was not clearly observed in other studies. Song et al. [31] compared the efficacies of 25, 50, 100, and 200 µg of DNA vaccine pcDNA-TA4-IL-2 of E. tenella, and founded that 25 µg was most effective in homologous challenge. Among chickens immunized intramuscularly with pMP13 expression vector ranging from 5 to 100 µg, those in both 5 µg and 50 µg DNA groups appeared to have the least oocyst discharge after challenge with E. acervulina, while those with the highest dosage (100 µg) group had the smallest oocyst discharge [32]. However, in our research, the median dosage group showed the best immune response, and a positive dose-response relationship was not observed. This is similar to those reported by others [31-33]. The reason for such a dose-response relationship is unclear. Further studies are needed to elucidate the mechanism.
In conclusion, our study indicated that the DNA vaccine encoding Gam56 protein could provide protection against E. maxima challenge. This investigation was of interest for the understanding of DNA vaccine protection and practical application. However, its immune mechanisms and optimal immune procedure in the field should be explored further. Further research is also required to develop multiple epitopic DNA vaccines or vaccine co-expressing cytokines to improve the immunizing efficacy in prevention of avian coccidiosis.
ACKNOWLEDGMENTS
This work was financially supported by the Project Funded by Key Lab for Avian Preventive Medicine of Jiangsu Province (grant no. K100014), Natural Science Foundation of Jiangsu Province (grant no. BK2009701), the Project Funded by the Priority Academic Program Development of Jiangsu Higher Education Institutions and the Program for Changjiang Scholars and Innovative Research Team in University (grant no. IRT0978). The authors thank Dr. Huigang Shen at Kansas State University for revising the manuscript.