New Molecules in Babesia gibsoni and Their Application for Diagnosis, Vaccine Development, and Drug Discovery
Article information
Abstract
Babesia gibsoni is an intraerythrocytic apicomplexan parasite that causes piroplasmosis in dogs. B. gibsoni infection is characterized clinically by fever, regenerative anemia, splenomegaly, and sometimes death. Since no vaccine is available, rapid and accurate diagnosis and prompt treatment of infected animals are required to control this disease. Over the past decade, several candidate molecules have been identified using biomolecular techniques in the authors' laboratory for the development of a serodiagnostic method, vaccine, and drug for B. gibsoni. This review article describes newly identified candidate molecules and their applications for diagnosis, vaccine production, and drug development of B. gibsoni.
INTRODUCTION
Babesia gibsoni is an intraerythrocytic apicomplexan parasite that causes piroplasmosis in dogs. The organism was recognized in India in 1910, and since, has been reported in Asia, Northern and Eastern Africa, Brazil, Europe, and even Australia [1,2,3]. This disease is mainly transmitted naturally by ticks, but many reports have demonstrated transmission by dog bites and blood transfusions and transmission via the transplacental route to the developing fetus [4,5,6,7]. B. gibsoni infection occurs frequently in dogs, and recently has become a serious problem from a clinical viewpoint, because the acute form of the disease typically results in serious clinical problems, such as, fever, thrombocytopenia, regenerative anemia, splenomegaly, and sometimes death [8,9]. Furthermore, infected animals may become chronic carriers and transmit the disease via ticks to other animals. Therefore, in order to control B. gibsoni infection, rapid, accurate diagnosis followed by prompt effective treatment, and the prevention of chronic carriers are needed.
Apart from clinical signs, microscopic examination is the simplest and most accessible diagnostic test for most veterinarians, and is reasonably sensitive for detecting intraerythrocytic parasites in Giemsa-stained blood smears during acute infection [10]. Moreover, microscopic examination is the only viable option available to veterinarians in many developing countries where babesiosis is endemic. However, the diagnosis of babesiosis in chronically infected and carrier dogs remains a significant challenge due to very low, often intermittent parasitemia [9]. Indeed, failure to detect parasites in animals with hemolytic anemia or thrombocytopenia has led to incorrect diagnoses in several documented cases [5,11].
PCR provides an alternative diagnostic test with good sensitivity and specificity [12,13,14,15]. Since parasite morphology is a poor guide to speciation, modified PCR techniques have been utilized to differentiate piroplasm species rapidly. For example, PCR-restriction fragment length polymorphism (RFLP) and nested PCR have been reported to differentiate B. canis subspecies and B. gibsoni in Australia and in endemic regions [16,17]. In addition, loop-mediated isothermal amplification (LAMP) has been reported to have the advantages of speed and specificity over nested PCR for the detection of B. gibsoni infection [18], and real-time PCR has been shown to enable the quantification of pathogen levels in blood and tissue samples [13]. Furthermore, a number of these PCR methods have been applied to filter-paper technologies, such as, FTA (Whatman Bioscience) and IsoCode Stix (Schleicher and Scheull) cards to facilitate the transport of samples to distant laboratories. Although the sensitivities of PCR methods continue to improve, access to molecular techniques for routine clinical diagnosis is still restricted to relatively few clinics worldwide, and these methods will not detect target DNA when there are no organisms in a sample. In addition, "false negative" results may be encountered during the chronic disease stage, and it is critical that this limitation is appreciated during the screening of asymptomatic dogs as potential blood donors and of potential carriers [19]. The ability of PCR to detect parasite DNA in chronically infected dogs can be improved by testing on more than one occasion, but complementary serological testing is advisable [20,21].
Immunofluorescent antibody test (IFAT) is the most widely used serological diagnostic test used for babesiosis [22,23,24]. However, its poor specificity due to cross-reactions between B. gibsoni and other closely related parasites with B. gibsoni, operator subjectivity, and its unsuitability for large-scale screening limit its use. ELISA is a more sensitive technique than IFAT and is appropriate for testing large number of samples, especially in field surveys [25,26]. However, although ELISA tests are powerful tools for serological surveys, poor quality antigens and sometimes cross-reactions limit their applications [27].
After the accurate diagnosis of B. gibsoni infection, effective control and management measures, which include the treatment of infected animals and tick control, are essential. The treatment of B. gibsoni-infected dogs is challenging because it is difficult to eradicate the parasite completely. Various drug regimens improve clinical manifestations, but fail to achieve eradication as determined by follow-up. In particular, clindamycin reduces parasitemia and the clinical symptoms of Babesia infection [28], but does not eradicate the parasite. In an experimental trial of atovaquone, parasite clearance and concomitant clinical symptom resolution were observed in a B. gibsoni-infected dog after 2 days of atovaquone treatment, but the parasite reappeared at ~1 month after last treatment [29]. In one study, atovaquone and azithromycin were found to reduce parasitemia but not to eliminate the parasite; furthermore the development of drug resistance was suspected [30].
Because eradication of the parasite is not an option using available drugs, a vaccine against B. gibsoni provides another possible control strategy. Unfortunately, no commercially vaccine is available for B. gibsoni, despite the efforts of several researchers. In a study by Sunaga et al., no detectable parasitemia was observed after B. gibsoni challenge in dogs inoculated with parasites attenuated by irradiation and serially passage in vitro [31,32]. However, the instability in vitro culture systems and the low parasitemia of B. gibsoni hamper the commercialization of such vaccines.
Therefore, in this review, we introduce some of the candidate molecules produced in the authors' laboratory for 1) diagnosis of B. gibsoni infection, 2) development of vaccines against the parasite, and 3) identification of potential drug targets.
IDENTIFICATION OF GENES ENCODING NEW PROTEINS
Since B. gibsoni is a eukaryotic organism, its genome contains untranslated introns. Furthermore, the complete genome sequence of B. gibsoni has not been determined, and thus, it is not possible to predict the locations of introns and exons, to amplify specific genes without introns by PCR, or to clone and express recombinant proteins for diagnostic, antigenic, or developmental purposes. Alternatively, the generation of complementary DNA (cDNA) from mRNA transcripts lacking introns provides a means of obtaining genes encoding novel candidate proteins in B. gibsoni for a development of diagnostic method, vaccine, and drug. In this regard, a full-length cDNA library was prepared from canine erythrocytes infected with the NRCPD strain of B. gibsoni using the vector-capping method [33,34].
In order to isolate high antigenic proteins or proteins with a conserved domain, a cDNA expression library constructed from B. gibsoni mRNA was used for immunoscreening. A number of potent antigens have been isolated using this immunoscreening technique, and their diagnostic and vaccine potentials subsequently demonstrated (Table 1) [34,35,36,37,38,39,40,41,42,43,44,45,46,47,48,49,50,51,52,53,54,55,56,57]. In parallel to the immunoscreening strategy, expressed sequence tag (EST) databases can be used to isolate and identify novel genes for developmental purposes. In our laboratory, the ESTs were mainly used to isolate drug targets for B. gibsoni infection after bioinformatics analysis to determine functional motifs, and experimental studies on parasites demonstrated their potencies as drug targets. Recently, several drug candidates of B. gibsoni, 2-Cys peroxiredoxin, leucine aminopeptidase, inosine 5'-monophosphate dehydrogenase, and dihydrofolate reductase-thymidylate synthase have been reported (Table 1) [58,59,60,61]. On the other hand, ESTs can be used to mine antigenic proteins by using bioinformatics software to predict the antigenicity (T- or B-cell epitope) and solubility of protein candidates for vaccine and serodiagnostic method development [62,63,64].
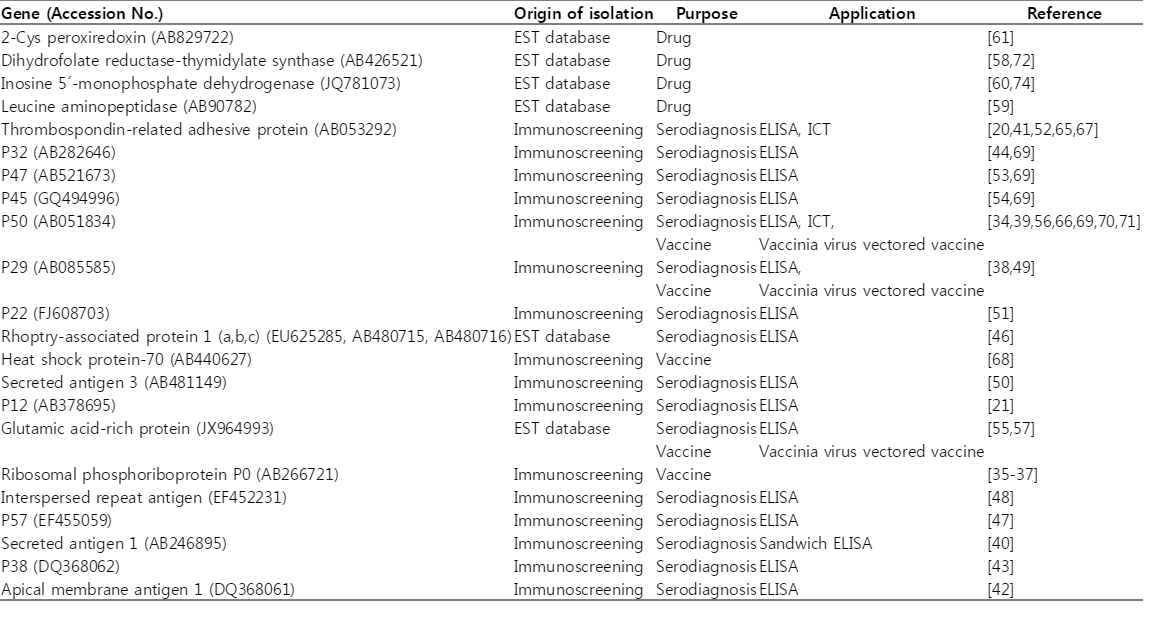
List of genes identified for possible diagnostic, vaccine, or drug use for Babesia gibsoni infection
After selecting a target gene by immunoscreening or bioinformatics analysis of ESTs, genes were amplified from the cDNA library or total mRNA of B. gibsoni by PCR or RT-PCR, respectively, using specifically designed primers with restriction enzyme sites in our laboratory. However, to improve the solubility and expression efficacy of recombinant proteins in the expression system, sequences with hydrophobic characteristic or low antigenicity were truncated. In the event, this did increase the solubility and expression of certain recombinant proteins [65,66].
APPLICATION OF RECOMBINANT PROTEINS
Serodiagnostic methods
Prior to using purified recombinant proteins for serodiagnosis, their antigenicities were evaluated by Western blotting using a canine serum infected with B. gibsoni. Proteins that reacted with B. gibsoni-infected serum were selected and then applied to develop serodiagnostic methods, such as, ELISA or ICT, with the sera from dogs experimentally and naturally infected with B. gibsoni.
ELISA
We performed indirect ELISA or double-antibody sandwich ELISA with the recombinant antigen of B. gibsoni to detect antibodies against the parasite. Among ELISAs using the identified antigens in our laboratory so far, ELISA with recombinant BgTRAP showed best performance at detecting antibody against B. gibsoni with high specificity and sensitivity [20,67]. However, recombinant BgTRAP was difficult to express in large amounts in the E. coli system. Thus, in order to increase expression efficacy, full-length BgTRAP was truncated and expression was optimized by adding ethanol, reducing the concentration of IPTG concentration, and culturing at lower temperature [65]. For sandwich ELISA, BgSA1 was selected and the sandwich ELISA could detect circulating BgSA1 in the plasma of dogs experimentally infected with B. gibsoni when parasitemia is 0.2% [40].
Immunochromatographic test (ICT)
ICT is a rapid, simple, and easy method in which the diagnosis is completed within 15 min after dropping a serum sample to the test strip. Therefore, another serodiagnostic method used to evaluate the expressed recombinant antigens of B. gibsoni is the ICT, in which recombinant truncated BgP50 (BgP50t), BgSA1, or truncated BgTRAP (BgTRAPt) are used to detect antibodies to B. gibsoni [39,45,52]. These ICTs with respective recombinant antigen were found to be specific, and not to react with serum from specific pathogen-free dogs. Furthermore, these antigens did not cross-react with sera from dogs experimentally infected with closely related parasites, such as, B. canis canis, B. canis vogeli, and B. canis rossi, or with N. caninum, and L. infantum. In contrast, the test did identify dogs experimentally infected with B. gibsoni and clinically infected dogs. We found that ICT using these antigens to be rapid, simple, accurate, and suitable for use at clinical sites for the diagnosis of B. gibsoni infection in dogs. Favorable results, that is, a sensitivity of 100% and a specificity of 93.78%, were reported when the test was performed using BgTRAPt for the detection of antibodies against B. gibsoni.
Vaccine development
No effective commercial vaccine against B. gibsoni is available. Recent strategies used for vaccine development are based on the use of parasite recombinant antigens or specific DNA rather than crude parasite lysates. In studies of B. gibsoni, although in vitro culture was available, parasitemia did not usually reach 5%, and thus, vaccine development focused on identifying high antigenic vaccine candidates (usually recombinant antigens) [35,36,37,49,66,68].
A gene encoding a 50-kDa surface protein (BgP50) of B. gibsoni was identified by immunoscreening using a cDNA library of B. gibsoni and serum from a dog experimentally infected with the parasite [34,69]. Once the gene had been identified, it was expressed in insect cells using baculovirus, and the obtained recombinant antigen was evaluated for antigenicity [66,70]. Surface proteins of parasite are ideal candidates for vaccine development because these proteins are the main targets of host immune responses during the host-parasite interaction. Accordingly, the surface antigens of parasites are logical targets for subunit vaccines. We produced a truncated form of BgP50 (BgP50t) without its C-terminal hydrophobic region, and found that recombinant BgP50t (rBgP50t) was secreted into the supernatant of insect cells infected with recombinant baculovirus. rBgP50t induced high antibody titers against the B. gibsoni merozoite when inoculated into mice, and antiserum to rBgP50t significantly inhibited B. gibsoni growth, indicating that rBgP50t might be a useful vaccine candidate. This inhibitory effect has been reported in a Severed Combined Immunodeficiency (SCID) mouse model transfused with canine erythrocytes [71]. Although the study focus of above-mentioned researches was on antibody-mediated immune response, it is vital to note that cellular immune responses could also play a role during B. gibsoni infection. Consequently, in future studies the antibody-mediated and cellular immune responses of the candidate vaccine antigens should be confirmed for full protection against B. gibsoni infection.
In another study, recombinant vaccinia virus-vectored plasmids were constructed to express rBgP50, rBgP29, or rBgGARP, respectively, and these vaccinia virus-vectored plasmids were used to immunize dogs [49,56,57]. The immunized dogs were shown to develop a high level of specific antibodies against the antigens as compared with non-immunized dogs. Furthermore, the antibody level was increased by booster immunization with a recombinant vaccinia virus. Additionally, the prime-boost immunization dose is known to provoke a specific IgG2 antibody response and IFN-r production in dogs. However, when the immunized dogs were challenged with B. gibsoni parasites, only partial protection was afforded by this immunization regime.
Ribosomal phosphoprotein P0 of B. gibsoni in its recombinant form (rBgP0) was evaluated as a universal vaccine candidate for B. gibsoni, B. microti, and B. rodhaini infections [36]. rBgP0 induced immunological response in mice against B. microti challenge [37]. In addition, the same rBgP0 showed cross-protective immunity against a lethal B. rodhaini infection in mice, and this cross-protection was mainly due to complement component 3 (C3) [35]. It is believed that antibody responses and cytokine production might play a role in this C3 mediated cross-protective immunity. In addition, heat shock protein-70 of B. gibsoni showed a cross-protective effect in mice infected with B. microti, and a partial protective effect was observed against B. microti infection [68].
Despite all these research attempts to produce candidate vaccines against B. gibsoni infection based on recombinant antigens, it has not been possible to identify an antigen that offers sufficient protection to justify its use as a commercial subunit vaccine. Nevertheless, studies on mechanisms of immune response during infection with B. gibsoni could provide new insight and lead to the discovering of candidate vaccines.
Drug development
Currently available drugs, including clindamycin, atovaquone, and azithromycin, reduce parasitemia and the clinical symptoms of B. gibsoni-infection, but do not eradicate the parasite [28,29,30]. These drugs were discovered by random screening of the anti-babesial activity of chemical compounds against other pathogens, not by the rational identification of drug targets. Although some metabolic pathways of B. bovis have been predicted from the whole genome sequences of B. bovis, knowledge regarding the biochemistry of Babesia parasites, including B. gibsoni, is limited.
Given the situation that the whole genome sequence of B. gibsoni is not available, the EST strategy provides an alternative means of identifying drug target by aligning ESTs with genes of other apicomplexans using BLASTX. Using this strategy, 2-Cys peroxiredoxin, inosine 5'-monophosphate dehydrogenase, dihydrofolate reductase-thymidylate synthase, and leucine aminopeptidase of B. gibsoni were identified and studied as drug targets [58,59,60,61]. The proteins were used for biochemical characterization and antiserum preparation against the respective recombinant proteins to study endogenous native proteins in B. gibsoni parasites. Those 4 proteins were found in the cytoplasm in B. gibsoni in an IFAT confocal microscopy based study [58,59,60,61]. Subsequently, the enzyme activities of the recombinant enzymes were determined using in vitro enzymatic assays.
In the case of dihydrofolate reductase-thymidylate synthase of B. gibsoni (BgDHFR-TS), a well-validated antifolate drug target in certain pathogenic apicomplexans, the catalytic activity of the recombinant enzyme was confirmed, and its kinetic parameters and inhibition by novel or known compounds were assayed. GST fused and non-fused recombinant BgDHFR-TS enzyme displays catalytic activity and had Km values similar to corresponding recombinant DHFR-TS in P. falciparum. In the same study, it was demonstrated that the inhibitory effect of 3 antifolates, methotrexate, pyrimethamine, and trimethoprim, on the catalytically active recombinant DHFR-TS enzyme of B. gibsoni paralleled their inhibitions of parasite growth in vitro [58]. Furthermore, methotrexate, which is a more-potent inhibitor of the BgDHFR-TS enzyme activity than pyrimethamine or trimethoprim, is also a potent inhibitor of B. gibsoni proliferation in vitro. Because the chemical structures of these antifolates resemble that of the substrate, dihydrofolate, the compounds are believed to compete with the substrate for binding to amino acid residues in the hydrophobic pocket of active site of recombinant BgDHFR enzyme [58,72].
Inosine 5'-monophosphate dehydrogenase (IMPDH) is an attractive drug target in the purine pathway because parasites rely on this pathway to meet their purine demands for nucleic acid synthesis [73]. Its enzyme activity was confirmed by measuring Km and IC50 values. Subsequently, mycophenolic acid, a structural analog of the cofactor NAD+, showed an inhibitory effect on the enzyme activity of BgIMPDH and on the growth of B. gibsoni, which indicated mycophenolic acid might inhibit the replication of B. gibsoni by targeting IMPDH of the purine pathway [60]. In addition, several IMPDH inhibitors, including mycophenolate mofetil, mizoribine, ribavirin, 7-nitroindole, and mycophenolic acid, were tested in in vitro cultures of B. gibsoni, and mycophenolate mofetil showed the most potent inhibitory effect on parasite growth [74].
Two recombinant proteins, that is, 2-Cys peroxiredoxin and leucine aminopeptidase, of B. gibsoni were produced, and subsequently, respective native proteins were identified in the cytoplasm of B. gibsoni. 2-Cys peroxiredoxin is an antioxidant enzyme that controls redox balance and by so doing protects membrane lipids, nucleic acids, and proteins from damage by reactive oxygen species (ROS). Antioxidant activity was confirmed using recombinant 2-Cys peroxiredoxin of B. gibsoni, which suggests that 2-Cys peroxiredoxin controls redox balance in B. gibsoni [61]. In addition, the enzyme activity of recombinant leucine aminopeptidase of B. gibsoni (rBgLAP) was confirmed using a leucine substrate. Although the substrate profile was slightly different from its homologue in P. falciparum, bestatin, an aminopeptidase inhibitor, showed similar inhibitory effect to enzyme activity of rBgLAP and its homologues in P. falciparum [59]. Few studies have been conducted on identification of drug targets and on the metabolic pathways of B. gibsoni to date, and thus, undiscovered metabolic pathways could yield possible drug targets. For example, apicoplast metabolism was recently examined as drug target in Plasmodium species, but this has not been reported to be present in B. gibsoni.
CONCLUSION
In this review, we introduce molecules identified and characterized for serodiagnosis and vaccine development and molecules that are drug targets for B. gibsoni infection. Of the identified antigens, a truncated recombinant BgTRAP produced promising results with high sensitivity and specificity for the detection of antibody specific to B. gibsoni by ELISA and ICT. For the next step, the BgTRAP could be used to develop a rapid diagnostic test of B. gibsoni infection using monoclonal antibodies specific to BgTRAP. The diagnostic test using the monoclonal antibodies would detect the antigen (BgTRAP) and by doing so differentiate dogs in current infection from past infection. In vaccine studies, parasitemia was only found to be partially reduced in studies of candidate vaccines, despite the induction of immune responses against B. gibsoni parasites in experimental animals. This could be improved by using effective adjuvants with the identified vaccine candidates based on better understanding of host immune responses induced by B. gibsoni. Regarding drug studies, few molecules have been identified to date, and the knowledge of metabolic pathways of B. gibsoni is still very limited. Therefore, further research is needed to identify the metabolic pathways that could be used to control this disease.
Notes
We have no conflict of interest related with this work.