Antifilarial and Antibiotic Activities of Methanolic Extracts of Melaleuca cajuputi Flowers
Article information
Abstract
We evaluated the activity of methanolic extracts of Melaleuca cajuputi flowers against the filarial worm Brugia pahangi and its bacterial endosymbiont Wolbachia. Anti-Wolbachia activity was measured in worms and in Aedes albopictus Aa23 cells by PCR, electron microscopy, and other biological assays. In particular, microfilarial release, worm motility, and viability were determined. M. cajuputi flower extracts were found to significantly reduce Wolbachia endosymbionts in Aa23 cells, Wolbachia surface protein, and microfilarial release, as well as the viability and motility of adult worms. Anti-Wolbachia activity was further confirmed by observation of degraded and phagocytized Wolbachia in worms treated with the flower extracts. The data provided in vitro and in vivo evidence that M. cajuputi flower extracts inhibit Wolbachia, an activity that may be exploited as an alternative strategy to treat human lymphatic filariasis.
INTRODUCTION
Lymphatic filariasis is one of the most neglected mosquitoborne diseases. It affects more than 150 million people in tropical and subtropical regions, and is caused by parasitic filarial nematodes [1-4]. It has high morbidity and heavy socioeconomic burdens [5,6]. The drugs frequently used to manage lymphatic filariasis include albendazole, a benzimidazole derivative; diethylcarbamazine, a derivative of piperazine; and ivermectin, which is believed to be a broad-spectrum anti-parasitic agent [7]. However, these drugs are effective mostly against microfilariae, but have limited activity against adult worms, which cause the disease [8]. In addition, the drugs elicit systemic and inflammatory side effects [5] and other medical complications [7]. Hence, alternative therapies have been sought through innovative and extensive phytomedicinal research that relies on traditional medicine [3].
Melaleuca cajuputi (Gelam tree), is used as a traditional medicine to treat cholera, muscular pain, diarrhea, scabies, and intestinal worms [9]. It has anti-inflammatory [10], anti-microbial [11], anti-cancer [12], and hepatoprotective [13] activities. A more recently adopted strategy to treat filarial infection is to target Wolbachia, an intracellular bacterial symbiont, the numbers of which increase dramatically (up to 600-fold) and peak shortly after infection of a mammalian host [14]. Filariasis control by targeting Wolbachia has been successfully demonstrated in humans using the antibiotics doxycycline and rifampicin, which inhibit embryogenesis and induce long-term sterility in the adult worm [7,15]. Antibiotics also inhibit the molting process of both third (L3) and fourth (L4)-stage larvae [14,16]. However, antibiotic regimens are lengthy and are contraindicated for children under 8 years old and for pregnant or breast-feeding women. Therefore, alternative drugs active against Wolbachia and without these contraindications will have significant impact. In this study, we present in vitro and in vivo evidence that methanolic extracts of M. cajuputi flowers inhibit Wolbachia and is active against Brugia pahangi worms.
MATERIALS AND METHODS
Chemicals
Roswell Park Memorial Institute medium (RPMI 1640 with L-glutamine), PBS (pH 7.2), fetal bovine serum (FBS), HEPES, 3-(4,5-dimethylthiazol-2-yl)-2,5-diphenyltetrazolium bromide (MTT), dimethylsulfoxide (DMSO), tetracycline, and ivermectin were purchased from Sigma (St. Louis, Missouri, USA).
Plant materials
Flowers of Melaleuca cajuputi were collected in September 2013 from Kedah State, in the northwestern part of the Malaysian peninsula. Identity was confirmed at the Herbarium of Rimba Ilmu, Institute of Biological Sciences, University of Malaya, Kuala Lumpur with the voucher no. KLU048231.
Flower extracts
M. cajuputi flowers were washed with distilled water, ground to powder, and dried in the dark for 7 days. Several independent extracts were obtained by macerating 100 g dried powder for 72 hr at room temperature in 1 L absolute methanol. Extracts were filtered into a Schott bottle through Whatman (No. 1) filter paper. The residue was macerated and filtered in the same manner another 2 times. Extracts were then pooled, concentrated in vacuo at 40˚C using a rotary evaporator, and finally dried for 3 hr under high vacuum to remove residual solvent.
Sample preparation
M. cajuputi flower extracts, ivermectin, and tetracycline were dissolved in pre-filtered DMSO to final concentrations of 10 mg/ml, 1 mg/ml, and 1 mg/ml, respectively. The antibiotics were then diluted in PBS (pH 7.2) to obtain 25 µg/ml ivermectin and 40 µg/ml tetracycline. Flower extracts were serially diluted 2-fold to prepare working concentrations between 1,000 µg/ml and 62.5 µg/ml for use against worms, and 10-50 µg/ml for use in Aa23 cells.
Anti-Wolbachia activity in Aa23 cells
Cell culture: Aedes albopictus Aa23 cells were derived from a culture generously provided by Prof. Scott O’Neill at Monash University, Clayton, Australia. Cells were cultured according to published methods [17]. Briefly, cells were grown at 26˚C in 25 cm2 flasks containing 5 ml medium consisting of equal parts of Mitsuhashi-Maramorosch and Schneider’s insect medium (Invitrogen, Carlsbad, California, USA) and supplemented with 20% heat-inactivated fetal bovine serum (Invitrogen). Cells were passaged every 3-4 days to prevent overgrowth. Triplicate cultures were used for each treatment.
Treatment with flower extracts: M. cajuputi flower extracts were dissolved at 1 mg/ml in DMSO, filter-sterilized, and stored in aliquots at -20˚C. Cells were treated in triplicate with 10-50 µg/ml extracts, 40 µg/ml tetracycline, or DMSO. Untreated cultures were used as the positive control. Media were replaced every 3 days with fresh media supplemented with fresh treatment. Cells were harvested after 7 days by centrifugation at 14,000 rpm for 10 min in 15 ml conical tubes, and resuspended in 1 ml fresh medium.
DNA Extraction
Total DNA was extracted from the cells using QIAamp Kit (Qiagen, Hilden, Germany), following the manufacturer’s instructions. DNA concentration and quality were determined by measuring absorbance on a Nanodrop ND-1000 spectrophotometer. Nucleic acid purity was determined by the ratio of absorbance at 260 nm to 280 nm (~1.8), and to 230 nm (1.8-2.2). Samples with ratios significantly different from these values (±0.4) were discarded.
Detection of Wolbachia by PCR
The presence of Wolbachia in Aa23 cells was verified by PCR using primers specific for the 590 fragment of Wolbachia surface protein [18,19]. The forward and reverse primers had sequence 5ʹ-TGGTCCAATAAGTGATGAAGAAAC-3ʹ and 5ʹ-AAAA ATTAAACGCTACTCCA-3ʹ, respectively. Primers for 28S nuclear ribosomal DNA (28sF3633/28sR4076) [20] were used as the control for template quality.
Each reaction was 20 µl in total volume, and contained 200 µM dNTPs, 300 nM primers, 0.5 U AmpliTaq DNA polymerase, 1×PCR buffer, 1.5 mM MgCl2, and 1 µl template. Reactions were denatured at 94˚C for 10 min, and then amplified over 35 cycles at 94˚C for 1 min, 55˚C for 1 min, and 72˚C for 1 min with final extension at 72˚C for 7 min. Amplification products (10 µl) were electrophoresed on 2% agarose, and stained with SYBR. Negative controls containing DNA-free water as template were used to test potential contamination in reaction components, while DNA from untreated Aa23 cells was used as the positive control.
Ethics statement
Experimental animal models were used according to guidelines approved by the Ethics Committee for Animal Experimentation, Faculty of Medicine, University of Malaya, Malaysia, Ethics no. PAR/21/11/2011/ZMN (R).
Isolation of infective larvae
Conditions were first optimized to obtain high yields of infective B. pahangi larvae from healthy, laboratory-bred Aedes togoi mosquitoes, eggs of which are maintained in the insectarium of the Department of Parasitology, Faculty of Medicine, University of Malaya. Thus, a filter paper with A. togoi eggs was immersed in a bowl containing 0.85% NaCl, and incubated at 37˚C to accelerate hatching. Hatched larvae were allowed to develop into pupae within 10 days, which were then transferred into an enclosed plastic jar containing clean water, and allowed to grow into adult mosquitoes within 2-3 days. Adult mosquitoes were then collected between 7-10 days and bloodfed on a naturally parasitized cat found in Pulau Carey, Selangor, Malaysia. B. pahangi infective stage larvae (L3) were collected from mosquitoes 10-14 days post-feeding using the Baermann’s technique [17].
Experimental infection and isolation of adult parasites from animal hosts
Male gerbils (Meriones unguiculatus) were intraperitoneally inoculated with 150 infective L3 larvae isolated from A. togoi mosquitoes. Infected animals were fed rodent chow and water. Adult worms for in vitro studies were obtained from the peritoneal cavity by necropsy 4-6 months after infection. Contaminants were removed from worms by washing 3-4 times in RPMI 1640 medium containing 20 mM HEPES, 100 µg/ml penicillin and 100 U/ml streptomycin. On the other hand, microfilariae were obtained by passing the peritoneal suspension through a 5 µm filter membrane (Millipore), and resuspending in RPMI 1640 medium.
Effect of flower extracts on microfilarial release, worm motility, and viability
The antifilarial activity of M. cajuputi flower extracts was evaluated in vitro by incubating adult worms for 7 days at 37˚C, 5% CO2, and 90% humidity in 4 ml RPMI medium supplemented with antibiotics, HEPES, 10% heat-inactivated FBS, and 6.25-1,000 µg/ml flower extracts. Antiparasitic activity was measured based on microfilarial release, worm motility, and viability.
The number of microfilariae released was determined in 1 ml medium on days 2, 4, and 6 post-treatment. Antifilarial activity is expressed as percent reduction in microfilariae released relative to the number released by untreated worms. Motility was measured daily by moving worms to fresh medium without antibiotics or flower extracts and observing under an inverted microscope. Mobility or death was scored according to a published scale [20], in which worms immobile for 10 sec were considered dead and scored 0, while slightly, moderately, and highly active worms were scored 1, 2, and 3, respectively. Treated worms were scored in comparison to the activity and motility of untreated worms. Results were reported as the mean motility score over 7 days. Data were collected in triplicate.
Worm viability was evaluated in duplicate after 7 days in culture using MTT (Sigma), as described by Saini and coworkers [5], with slight modification. After viability was assessed by microscopic examinations, worms were then transferred to a 24-well flat-bottomed plate, and incubated for 30 min in 0.5 ml PBS (pH 7.4) containing 0.5 mg/ml MTT. Subsequently, plates were incubated in the dark for 2 hr at 37˚C [17]. Formazan crystals formed were solubilized with occasional gentle shaking for 1 hr at room temperature in 100-200 µl DMSO. The absorbance at 492 nm of the resulting formazan solution was measured in a microplate spectrophotometer using DMSO as blank. Adult females treated with DMSO only were used as the positive control, while worms killed by heating at 56˚C for 30 min were used as the negative control. Percent inhibition of formazan production was estimated according to the following equation:
where T, C, and H are absorbance values obtained from treated, control, and heat-treated worms, respectively.
Transmission electron microscopy
Treated and untreated B. pahangi female worms were examined by transmission electron microscopy after 7 days in culture, following published protocols [16], with some modification. Briefly, worms were fixed for 2 hr at 25±1˚C with 3% glutaraldehyde in 0.1 M cacodylate buffer (pH 7.4). Samples were then treated with 1% osmium tetroxide in cacodylate buffer, dehydrated in increasing concentrations of ethanol (30-95%), and embedded in Eponate 812 low-viscosity resin (Ted Pella, Redding, California, USA). Ultrathin sections of 65-70 nm were obtained, stained with uranyl acetate and Reynold’s lead citrate, and examined on a Zeiss 912 Omega transmission electron microscope (Oberkochen, Germany).
Molecular analysis
DNA was extracted from worms using DNeasy Blood and Tissue Kit (Catalog no. 69504, Qiagen) following the manufacturer’s protocol. Wolbachia surface protein was amplified by PCR as described previously [21], with modifications. The forward and reverse primers had sequence 5ʹ-GCAGACGGGTGA GTAATGTATA-3ʹ and 5ʹ-GCCACTGGTGTTCCTCCTAATA-3ʹ. The reaction consisted of 40 cycles at 95˚C for 30 sec, 50˚C for 30 sec, and 72˚C for 60 sec. Newly developed worms were used as the positive control, and water was used as the negative PCR control. Amplification products from 6 worms in the same treatment regimen were pooled, separated on 1.5% agarose, and stained with SYBR Safe.
Statistical analysis
Data are reported as mean±SD of triplicate experiments and were analyzed in Prism (GraphPad Software, San Diego, California, USA). One-way ANOVA was used to test differences among treatment groups, and P-values <0.05 were considered significant.
RESULTS
In vitro effects of flower extracts against Wolbachia sp.
PCR amplification of Wolbachia surface protein generated a 590-bp band from Wolbachia-colonized Aa23 cells (Fig. 1A). The gene was totally lost in cells treated with 50 µg/ml flower extracts for 7 days, denoting anti-bacterial activity. Presumably, this effect was not due to general cytotoxicity, as MTT assays indicated that M. cajuputi flower extracts were not cytotoxic in Aa23 cells up to 200 µg/ml (data not shown). In addition, all DNA preparations produced a band at approximately 400 bp when amplified with primers for 28S ribosomal DNA, verifying template quality (Fig. 1B). Thus, the effects of flower extracts were investigated in vivo.
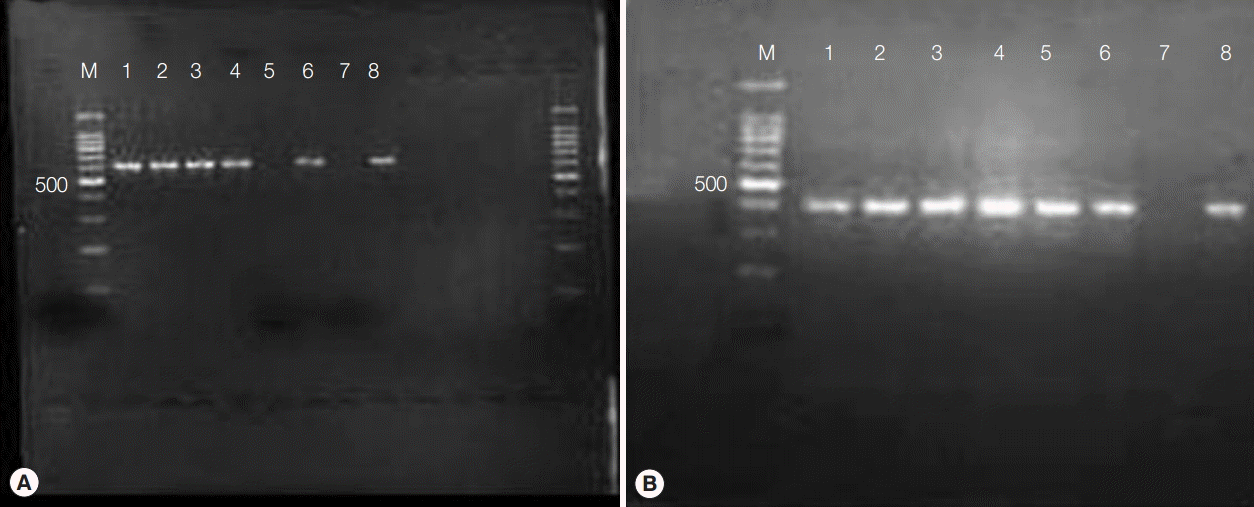
PCR amplification of Wolbachia surface protein (A) and 28S ribosomal DNA (B) in Aa23 cells treated with Melaleuca cajuputi flower extracts for 7 days. Lane M, 100 bp molecular ladder; lanes 1-5, cells treated with 10, 20, 30, 40, and 50 μg/ml flower extracts, respectively; lane 6, cells treated with tetracycline; lane 7, PCR using water as template; lane 8, untreated cells.
Effects of flower extracts against the filarial worm B. pahangi
Untreated female worms released microfilariae at comparable rates throughout 7 days in culture (Fig. 2). However, a progressive decrease in microfilariae was observed in worms treated with M. cajuputi flower extracts (Fig. 2). The decrease was dose-dependent, such that the release completely ceased at day 6 in worms treated with 1,000 µg/ml flower extract. These observations were in line with the effects of the reference drug tetracycline (Fig. 2).
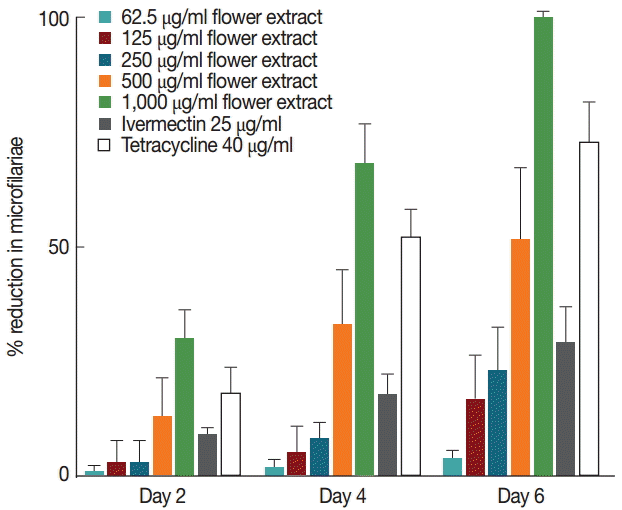
Percent reduction at days 2, 4, and 6 in microfilariae released by Brugia pahangi female worms treated with Melaleuca cajuputi flower extracts. Data are mean±SE.
As expected, there was no observable change in the motility of untreated worms, which had a constant motility score of 3 over 7 days. Notably, 62.5-500 µg/ml M. cajuputi flower extracts, tetracycline, and the reference drug ivermectin did not significantly reduce (P>0.05) the mean motility score. However, 1,000 µg/ml flower extract was found to cause complete immobility at days 6 and 7.
The effect of M. cajuputi flower extracts on worm viability was also evaluated using MTT (Fig. 3). In worms treated with 62.5-1,000 µg/ml flower extracts for 7 days, viability decreased significantly (P<0.05) in a dose-dependent manner, especially at concentrations above 500 µg/ml. In fact, 1,000 µg/ml M. cajuputi flower extracts reduced microfilarial release and worm viability more drastically than the standard reference drugs ivermectin and tetracycline at 25 µg/ml and 40 µg/ml, respectively (Fig. 3).
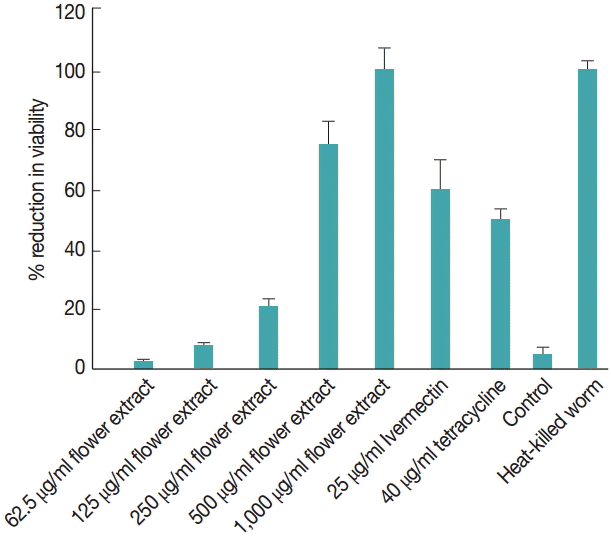
Percent reduction in the viability of adult Brugia pahangi worms treated with Melaleuca cajuputi flower extracts, as measured by MTT reduction assay. Untreated parasites were used as negative control, while the reference drugs ivermectin and tetracycline were used for comparison. Data are mean±SE. There is statistically significant difference among treatments (P<0.05).
Effects of flower extracts against Wolbachia endosymbionts in filaria adults
The activity of flower extracts against Wolbachia endosymbionts in adult worms was evaluated by amplifying Wolbachia surface protein, which has been reported to be about 590 bp [29,30]. A fragment of comparable size was amplified using DNA preparations from adult worms (Fig. 4). As in Aa23 cells, the amplicon diminished in dose-dependent fashion with exposure to M. cajuputi flower extracts, indicating antibacterial activity in vivo, especially at concentrations beyond 500 µg/ml.
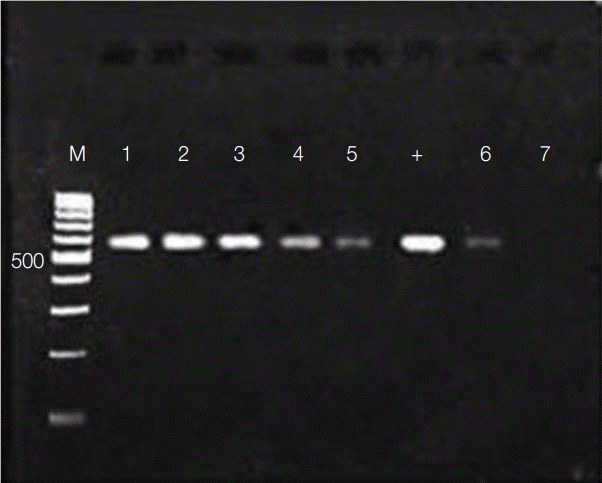
Agarose gel electrophoresis of Wolbachia surface protein (590 bp) amplified from female Brugia pahangi worms (n=6) treated with 62.5, 125, 250, 500, and 1,000 μg/ml Melaleuca cajuputi flower extracts (lanes 1-5) and tetracycline (lane 6). Newly developed adult worms (+) were used as the positive control, while water was used as the negative PCR control (lane 7).
Ultrastructural effects of flower extracts against Wolbachia endosymbionts
The effect of flower extracts on filarial parasites was further evaluated by transmission electron microscopy (TEM) (Fig. 5). Numerous intracellular Wolbachia cells were detected in the hypodermal cord of untreated worms (Fig. 5A). On the other hand, degraded Wolbachia cells were observed in worms treated with flower extracts (Fig. 5B) and tetracycline (Fig. 5C), along with phagocytized bacterial remnants in cellular vacuoles (black arrows).
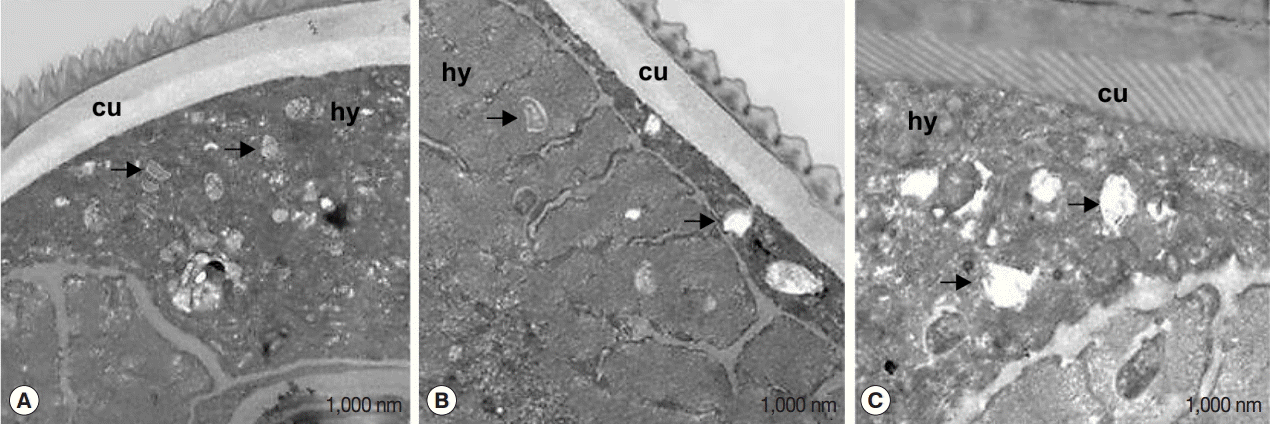
Transmission electron microscopy of Wolbachia in the hypodermis of untreated B. pahangi worms (A), or of worms treated with Melaleuca cajuputi flower extracts (B), and 40 μg/ml tetracycline (C). In (B), Wolbachia was reduced in abundance and appeared degenerated (arrow). In (C), the endosymbiont appeared dead, and vacuoles contained only remnants or membrane whorls (arrow). Scale bar=1,000 nm. cu, cuticle; hy, hypodermis.
DISCUSSION
Antibiotics against Wolbachia have been shown in extensive human trials to also induce loss of filarial worm fertility and viability. In line with these observations, our results in vitro and in vivo also indicated that M. cajuputi flower extracts reduced microfilarial release, worm motility, and viability by potently inhibiting Wolbachia, as indicated by the reduction in Wolbachia abundance and the total loss of the gene encoding Wolbachia surface protein. Anti-Wolbachia activity was further confirmed by the observation of degraded and phagocytized Wolbachia in worms treated with flower extracts. In turn, this antibacterial activity enhances antifilarial activity, as evidenced by the significant reduction in worm viability after 7 days of treatment. However, short-term treatment with flower extracts did not reduce Wolbachia to the same extent as tetracycline. Nevertheless, flower extracts and tetracycline at the highest doses reduced microfilariae release and worm motility more drastically than standard antiparasitic drugs.
Whereas untreated female worms released microfilariae throughout 7 days in culture, a progressive decrease in microfilariae was observed in worms treated with M. cajuputi flower extracts. The release completely ceased at day 6 in worms treated with 1,000 µg/ml flower extracts. These results were similar to the effects of the reference drug tetracycline, which was demonstrated by Gunawardena et al. [21]. It was of note that 1,000 µg/ml flower extracts were found to cause complete immobility of worms at days 6 and 7, which was consistent with microfilariae release and with earlier studies [22-27]. Our results were similar to published data describing the antifilarial activity of extracts from Bauhinia racemosa [28] and Hibiscus mutabilis [5] against B. malayi and Setaria cervi, respectively.
The activity of flower extracts against Wolbachia endosymbionts in adult worms was evaluated by amplifying Wolbachia surface protein of about 590 bp [29,30]. Our results indicated antibacterial activity in vivo, especially at concentrations beyond 500 µg/ml. Previous studies have demonstrated similar effects with tetracycline [29]. It is of note, however, that flower extracts did not reduce Wolbachia abundance to the same extent as tetracycline, presumably because the treatment regimen was too short. Indeed, tetracycline treatment for less than 2 weeks is insufficient to clear Wolbachia from Brugia worms [31].
When the effects were evaluated by transmission electron microscopy, numerous intracellular Wolbachia cells were detected in the hypodermal cord of untreated worms, whereas degraded Wolbachia cells were observed in worms treated with flower extracts and tetracycline, along with phagocytized bacterial remnants. The data are in line with reports of Wolbachia degeneration in B. malayi [14] and Onchocerca ochengi [31] treated with tetracycline. The total number of endosymbionts was generally lower in worms treated with flower extracts than in untreated worms, indicating anti Wolbachia activity. In turn, this antibacterial activity should enhance antifilarial activity, because the endosymbiont has been reported to be essential for development and fertility [32,33]. Notably, Wolbachia is known to elicit innate inflammatory reactions in the human host, and antibodies against its surface proteins are a key to the development of lymphedema and hydrocele in patients with bancroftian filariasis [32].
In conclusion, the data provide evidence that M. cajuputi flower extracts are active against both microfilariae and adult worms. The extracts significantly reduce microfilarial release and viability of adult worms by targeting endosymbiotic Wolbachia, as indicated by DNA and TEM analysis. However, mechanistic details remain unclear, and will be investigated in future studies.
Acknowledgements
This study was funded by the Ministry of Higher Education, Malaysia (FRGS FP011/2011A) and the University Malaya Research Grants (PPP) (PG085-2012B, RG313-14AFR, RG546-14HTM, and RG515-13HTM).
Notes
The authors have declared that no conflict of interests exists, financial or otherwise, from any company or other entities.