Signaling Role of Adipocyte Leptin in Prostate Cell Proliferation Induced by Trichomonas vaginalis
Article information
Abstract
Leptin is a type of adipokine mainly produced by adipocytes and reported to be overproduced in prostate cancer. However, it is not known whether it stimulates the proliferation of prostate cells. In this study, we investigated whether benign prostatic hyperplasia epithelial cells (BPH-1 cells) infected with Trichomonas vaginalis induced the proliferation of prostate cells via a leptin signaling pathway. To investigate the effect of crosstalk between adipocyte leptin and inflamed epithelial cell in proliferation of prostate cells, adipocytes 3T3-L1 cells were incubated in conditioned medium of BPH-1 cells infected with T. vaginalis (T. vaginalis-conditioned medium, TCM), and then the adipocyte-conditioned medium (ATCM) was identified to cause proliferation of prostate cells. BPH-1 cells incubated with live T. vaginalis released pro-inflammatory cytokines, and conditioned medium of these cells caused migration of adipocytes. When prostate stromal cells and BPH-1 cells were incubated with adipocyte conditioned medium containing leptin, their growth rates increased as did expression of the leptin receptor (known as OBR) and signaling molecules such as JAK2/STAT3, Notch and survivin. Moreover, blocking the OBR reduced this proliferation and the expression of leptin signaling molecules in response to ATCM. In conclusion, our findings show that inflamed BPH-1 cells infected with T. vaginalis induce the proliferation of prostate cells through leptin-OBR signaling. Therefore, it is likely that T. vaginalis contributes to prostate enlargement in BPH via adipocyte leptin released as a result of inflammation of the prostate.
INTRODUCTION
The protozoan parasite, Trichomonas vaginalis, is the cause of trichomoniasis, a common sexually transmitted disease [1]. The World Health Organization estimates that over half of the 143 million new T. vaginalis infections each year involve males [2]. The majority of infections in men are asymptomatic and remain undiagnosed and untreated, and are thought to result in persistent prostatic infection [3]. Trichomonas vaginalis has been detected in prostate tissue in prostate cancer and benign prostatic hyperplasia (BPH), as well as in the urine of patients with prostatitis [4–6]. We have shown that T. vaginalis infection causes acute and chronic inflammation within the parenchyma of the prostate gland [7], and prostate inflammation, including the production of cytokines, appears to be a cause of BPH and prostate cancer [8,9]. Inflammatory mediators are secreted by the prostatic stroma consequent upon aging, and the levels of these mediators are sufficient to increase the proliferative rate of both epithelial and stromal fibroblasts [10]. Although, inflammation may play a role in the development of benign proliferative diseases of the prostate such as BPH, the connections between inflammation, T. vaginalis infection and prostate enlargement are not fully understood.
Benign prostate hyperplasia (BPH) occurs clinically in about 50% of men over the age of 70 [11]. It is not clear what elicits BPH, but aging may be a prerequisite for its induction [12]. Recently, some reports have also highlighted the key roles of hormonal alterations, metabolic syndrome and obesity in the pathogenesis of BPH [13–16].
Obesity causes many physiological problems and can induce chronic inflammation [17]. In addition, infection by Neospora caninum, a protozoon, raises serum leptin levels and contributes to long-term inflammation in adipose tissue [18]. Adipocytes influence the endocrine system and immune responses through several cytokine-like mediators known as adipokines. Leptin is an adipokine originally identified as a key molecule in the regulation of food intake and body weight. It interacts with other hormones and energy regulators, mediating the effects of insulin, glucagon, insulin-like growth factor, growth hormone, glucocorticoids, cytokines, and metabolites [19]. Obesity is associated with several diseases, and many reports have suggested a link between leptin and cancer growth. Leptin also stimulates the growth of prostate, breast, lung, ovarian and pancreatic cancer cells [20–24]. BPH is closely linked to obesity, and abdominal obesity and serum leptin levels influence prostate growth [25] and are high-risk factors for clinical BPH [18]. However, the connection between BPH and obesity is not yet fully understood at the molecular level. Furthermore, it is not clear whether leptin influences the progression of BPH.
We showed previously that BPH epithelial cells that had been infected with T. vaginalis caused inflammation, and induced the proliferation of prostate stromal cells by activating of mast cells [26]. Subsequently, inflammatory mediators released from the proliferating stromal cells by T. vaginalis infection were shown to induce the proliferation of BPH epithelial cells [27]. However, it is not known how the crosstalk between T. vaginalis infection and adipocytes causes the proliferation of prostate cells. In particular, it is not known whether leptin released from the adipocytes is directly responsible for the proliferation of normal and benign prostate cells. To assess whether inflammation due to T. vaginalis infection in obese conditions is involved in the progression of BPH, we examined whether adipocyte, incubated with culture supernatants of BPH epithelial cells infected with T. vaginalis, induces the proliferation of prostate stromal and epithelial cells by production of leptin. Our findings suggest that BPH epithelial cells infected with T. vaginalis induce adipocyte migration and activation and that in turn, the adipocytes produce leptin, which stimulates the proliferation of prostate cells.
MATERIALS AND METHODS
Cultivation of parasite and host cells
Trichomonas vaginalis isolate T016, human BPH epithelial cell line (BPH-1 cell) and human prostate stromal cell line (WPMY-1 cell) were cultured as described in our previous study [26]. All the cells were incubated in 5% CO at 37°C.
Cultivation of adipocytes
Mouse preadipocyte cells (3T3-L1 cell) were a gift from Prof. Jae-woo Kim (Yonsei University). They were grown in DMEM containing 10% bovine calf serum (BCS, Rosedale, Auckland, New Zealand) and 1× penicillin-streptomycin solution. To induce differentiation, 2-day post-confluent 3T3-L1 cells were incubated in DMEM containing 10% FBS, 0.5 mM 3-isobutyl-1-methylxanthine, 1 μM dexamethasone, and 10 μg/ml insulin for 3 days. The cells were then cultured in DMEM containing 10% FBS and insulin for another 2 days, after which they were grown in DMEM containing 10% FBS. Lipid droplets in the cells were detected by oil-red-O staining [28].
Preparation of conditioned media
To probe the effect of interactions between adipocytes and inflamed epithelial cells on the proliferation of prostate cells, in vitro co-cultures of adipocytes and BPH-1 cells infected with T. vaginalis were established. Conditioned media were made as described previously [26]. Briefly, BPH-1 cells were seeded at 1×105 cells/well in complete medium in 12-well plates. After 24 hr, the medium was changed to serum-free medium and the cells were cultured for another 24 hr to stabilize them. They were then incubated with or without live T. vaginalis in a ratio of 1:10 for 6 hr and supernatants were collected, filtered through 0.2 mm filters, and stored frozen. The culture supernatants of the BPH-1 cells incubated with and without T. vaginalis were named T. vaginalis-conditioned medium (TCM) and conditioned medium (CM), respectively. Differentiated adipocytes were incubated with TCM and CM, in the same way, yielding the conditioned media ATCM and ACM. Final concentrations of the conditioned media used for various assays were adjusted to 10% with cell culture medium.
Measurement of cytokines
Cytokine concentrations in conditioned media were measured by ELISAs. Concentrations of cytokines IL-6, CXCL8, CCL2, and IL-1β were measured with human ELISA sets (BD Biosciences, San Jose, California, USA) and concentrations of adipokines CXCL1, IL-6, and CCL2 were measured with mouse ELISA sets (BD Biosciences). Leptin levels were measured with a mouse leptin ELISA kit (Abcam, Cambridge, Massachusetts, USA).
Preadipocyte chemotaxis assay
Chemotaxis was assessed using 24-well plates fitted with 5 mm pore polycarbonate membrane inserts. The chemotactic index was calculated as described in our previous study [28]. Briefly, the lower wells were filled with 350 ml of TCM, and polycarbonate membrane inserts were placed over the lower wells. For adhesion of the cells, the filters were pretreated with human plasma fibronectin (100 mg/ml) overnight at 4°C, and air-dried for 30 min. The upper wells were filled with 200 ml of preadipocyte cell (2×105 cells/well) in serum-free medium, and the plates were incubated for 24 hr at 37°C. After incubation, cells that did not migrate through the pores were wiped off the membranes with cotton swabs. The cells that had migrated to the underside of the membrane were fixed in methanol and stained with Giemsa stain solution, and 5 randomly-selected fields per membrane were counted under a light microscope. The chemotactic index was calculated from the number of cells that migrated relative to the control.
Proliferation and invasion assays
To measure cell proliferation, wound healing assay and BrdU incorporation assay were used. For wound healing assays, cultured prostate cells that had reached confluence were wounded by scraping across the surface of the well with a sterile micropipette tip. The cells were washed immediately and incubated in 10% ATCM for 48 hr. Before and after incubation, at least ten different fields of the wounded area of each sample were photographed with an inverted microscope (Leica, Las software) [26]. We used the 5-bromo-20-deoxyuridine (BrdU) incorporation assay to detect DNA synthesis following ATCM treatment of prostate cells. For the BrdU assay, prostate cells were seeded at 5×104/well on sterile cover glasses in 12-well plates, allowed to adhere overnight and cultured with 10% ATCM for 48 hr. During that time, BrdU (10 μg/ml) was added to the cells once a day. The cells were fixed for 10 min at −20°C, and denatured for 30 min at 37°C. For blocking and permeabilization, the cells were placed in blocking buffer for 1 hr at room temperature. They were then incubated with anti-BrdU antibody (Abcam) overnight at 4°C, and stained with Alexa 594-labelled goat anti-rabbit lgG (Invitrogen, Carlsbad, California, USA) at 37°C for 1 hr. They were mounted in Vectashield mounting medium (Vector Laboratories, Burlingame, California, USA) with DAPI to visualize nuclei. Fluorescence was measured with a fluorescence microscope (Leica, Las software). The invasiveness of prostate stromal cells treated with conditioned medium was tested according to the protocol described in our previous report [26].
Immunofluorescence detection of the leptin receptor
The leptin receptor is known as LEP-R or OB-R and has been used as OBR in several papers. To observe expression of the leptin receptor (OBR) on the surface of prostate cells, we used an immunofluorescence assay [26]. Transmembrane OBR, which belongs to the cytokine receptor superfamily, mediates the effects of leptin. Prostate cells were seeded at 2×105 cells/well on sterile cover glasses in 12-well plates and cultured in complete medium. After overnight incubation, the medium was changed to ATCM, and the cells were cultured at 37°C for 24 hr. They were then washed, fixed with 4% paraformaldehyde for 10 min at −20°C and blocked with 0.1% normal goat serum for 1 hr. The cover glasses were incubated with anti-OBR antibody (Abcam) overnight at 4°C, washed and stained with Alexa 594-labelled goat anti-rabbit lgG (Invitrogen) at 37°C for 1 hr. The cells were mounted in Vectashield mounting medium (Vector Laboratories) with DAPI to visualize nuclei. Fluorescence was measured with a fluorescence microscope (Leica, Las Software).
Reverse transcription PCR (RT-PCR) and quantitative real-time PCR (Q-PCR)
Levels of mRNA in T. vaginalis-treated BPH-1 cells and TCM-treated 3T3-L1 cells were measured by RT-PCR [26]. BPH-1 cells were seeded at 2×105 cells/well in 24-well plates in culture medium. After overnight incubation, the medium was changed to serum-free medium and then live T. vaginalis was added. In case of 3T3-L1 cells, cells were seeded at 5×104 cells/well on 24-well plates with the culture medium. The medium of adipocytes replaced to serum-free medium with 10% TCM. mRNA levels of leptin signaling molecules were examined by Q-PCR [26]. Prostate cells were seeded at 2×105 cells/well in 24-well plates with culture medium. After overnight incubation, the medium was changed to serum-free medium with 10% ATCM. Anti-OBR antibody was used to block leptin signaling. After reaction, the cells were collected and total RNA was extracted using Tri-RNA reagent (Favorgen Biotech Corp, Kaohsiung, Taiwan). RNA samples were reverse transcribed to cDNA using Maxime RT preMix (Oligo (dt) 15 Primer) (iNtRON Biotechnology, Sungnam, Korea), and the cDNA was used as template for subsequent PCR amplification using following gene-specific primers. Human CXCL8; 5′-GGCAGCTTCCTGATTTCTG-3′, 5′-CGCAGTGTGGTCCACTCTCA-3′, Human CCL2; 5′-CAAGCAGAAGTGGGTTCAGGA-3′, 5′-TCTTCGGAGTTTGGGTTTGC-3′, Human IL-6; 5′-TGGCTGCAGGACATGACAACT-3′, 5′-ATCTGAGGTGCCCATGCTACA-3′, Human IL-1β; 5′-CTGATGGCCCTAAACAGATGAAG-3′, 5′-GGTCGGAGATTCGTAGCAGCTGGAT-3′, Human JAK2; 5′-GAGCCTATCGGCATGGAATA-3′, 5′-ACTGCCATCCCAAGACATTC-3′, Human STAT3; 5′-TGTGCGTATGGGAACACCTA-3′, 5′-AGAAGGTCGTCTCCCCCTTA-3′, Human Notch1; 5′-GAGGCGTGGCAGACTATGC-3′, 5′-CTTGTACTCCGTCAGCGTGA-3′, Human Jagged1; 5′-TCGGGTCAGTTCGAGTTGGA-3′, 5′-AGGCACACTTTGAAGTATGTGTC-3′, Human Jagged2; 5′-AGCTGGACGCCAATGAGTG-3′, 5′-GTCGTTGACGTTGATATGGCA-3′, Human survivin; 5′-TCCACTGCCCCACTGAGAAC-3′, 5′-TGGCTCCCAGCCTTCCA-3′, Mouse leptin; 5′-TGCTGCAGATAGCCAATGAC-3′, 5′-GAGTAGAGTGAGGCTTCCAGGA-3′, β-actin; 5′-CCAGAGCAAGAGAGGTATCC-3′, 5′-CTGTGGTGGTGAAGCTGTAG-3′. Target mRNA was amplified with Maxime PCR PreMix (i-StarTaq) (iNtRON Biotechnology) using a Mastercycler (Eppendorf, Hamburg, Germany) for RT-PCR, and PCR products were separated by agarose gel electrophoresis. Bands were visualized with StaySafe Nucleic Acid Gel stain (RBC, Banqiao, New Taipei, Taiwan) and identified using an ExcelBand™ 100 bp+3K DNA Ladder (SMOBIO, Hsinchu, Taiwan). For quantitative real-time PCR, target mRNA was amplified using a LightCycler 480 SYBR Green I Master (Roche Life Science, Mannheim, Germany) and analyzed with the LightCycler 480 Software (Roche Life Science). β-actin was used as an internal control.
Western blot analysis
Cells were harvested and lysed in PRO-PREP protein extraction solution (iNtRON Biotechnology). Cell lysates were scraped, and protein concentrations were measured with the Bradford assay. Equal amounts of protein were denatured in 5×protein sample buffer (ELPIS biotech, Daejeon, Korea), separated by SDS-PAGE on 12% polyacrylamide gel, and transferred to Immun-Blot®PVDF membranes (Bio-Rad, Quarry Bay, Hong Kong). The membranes were blocked with 3% bovine serum albumin, and probed with the following primary antibodies overnight at 4°C: anti-leptin antibody (Abcam), anti-p-JAK2 antibody (Abcam), anti-p-STAT3 antibody (Cell signaling, Danvers, Massachusetts, USA), anti-Notch1 antibody (bioworld, Dublin, Ohio, USA), anti-NICD antibody (Abcam), anti-Jagged1 antibody (Abcam), anti-survivin antibody (Enzo Life Sciences, Farmingdale, New York, USA) or β-actin polyclonal antibody (Abcam). After washing, the membranes were incubated with goat anti-rabbit IgG antibody (H+L), HRP conjugated (Bioss Antibodies, Woburn, Massachusetts, USA) for 1 hr at room temperature, blots were visualized using Chemiluminescent Sensitive Plus HRP Microwell and/or Membrane Substrate (SurModics, Eden Prairie, Minnesota, USA) and signals were measured with a Chemi-Doc® (Bio-Rad, Hercules, California, USA) [26].
Statistical analysis
Statistical analyses were performed using SPSS statistical software, version 21 (IBM, Chicago, Illinois, USA). The Mann-Whitney U test was used to compare results, and 2 tailed P-values <0.05 were considered statistically significant. All data are expressed as means±SEMs of three to four independent experiments.
Ethical statement
This study was approved by the Institutional Review Board of Hanyang University Hospital (IRB No. 2019-04-011-002). Waiver of informed consent for this study was obtained from IRB based on the retrospective analyses of archived tissues and clinical data.
RESULTS
Cytokine production by BPH epithelial cells cocultured with Trichomonas vaginalis
We first examined cytokine production by BPH-1 cells incubated with T. vaginalis (1:10) for 6 hr. The BPH-1 cells incubated with T. vaginalis released cytokines IL-6 (448.8 pg/ml), CXCL8 (548.6 pg/ml), CCL2 (435.9 pg/ml) and IL-1β (147.5 pg/ml), and cytokine production was significantly higher than in the absence of T. vaginalis (P<0.05; Fig. 1A). Similarly, cytokine mRNA levels of cells incubated with T. vaginalis were higher than those incubated without T. vaginalis (Fig. 1B). T. vaginalis was confirmed to cause inflammatory response in BPH-1 cells. Culture supernatants of BPH-1 cells incubated with and without T. vaginalis were designated T. vaginalis-conditioned medium (TCM) and conditioned medium (CM), respectively. To identify lipid droplets accumulated in the cytoplasm of mature adipocytes, Oil-Red O staining was performed. Lipid accumulation of the adipocytes was observed in red (Fig. 1C).
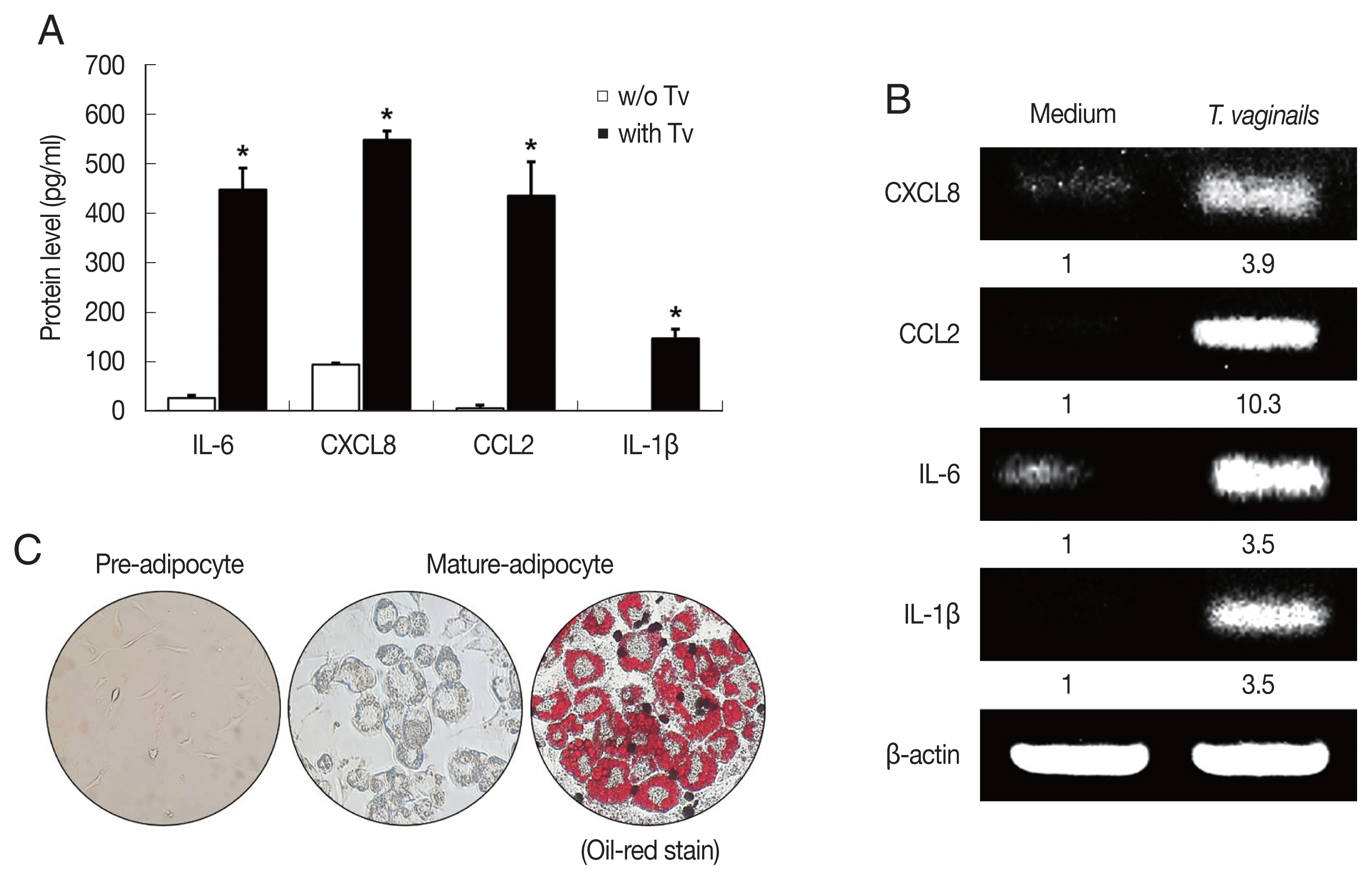
Cytokine production by benign prostate hyperplasia-1 (BPH-1) cells infected with Trichomonas vaginalis, and differentiation of preadipocytes (3T3-L1) into mature adipocytes. To examine cytokine production, BPH-1 cells were incubated with live T. vaginalis (Tv). Cytokines (IL-6, CXCL8, CCL2, and IL-1β) and their mRNAs were measured by ELISA (A) and RT-PCR (B), respectively. Medium, culture supernatant of BPH-1 cells not exposed to T. vaginalis. *P<0.05 versus w/o T. vaginalis. To identify lipid droplets accumulated in the cytoplasm of mature adipocytes, oil-Red O staining was performed. Lipid accumulation of the adipocytes was observed in red (C).
Migration of preadipocytes, and adipokine expression in adipocytes induced by TCM
Recently it was reported that pre-adipocytes are recruited preferentially to prostate cancer tissue rather than to surrounding normal prostate tissue [29]. We examined the effect of TCM on the migration of 3T3-L1 cells using a chemotaxis assay. Chemotaxis by 3T3-L1 cells exposed to TCM was significantly greater than by cells exposed to CM (P<0.05; Fig. 2A). This result suggests that factors released by inflamed BPH-1 cells infected with T. vaginalis play a role in the recruitment of 3T3-L1 cells.
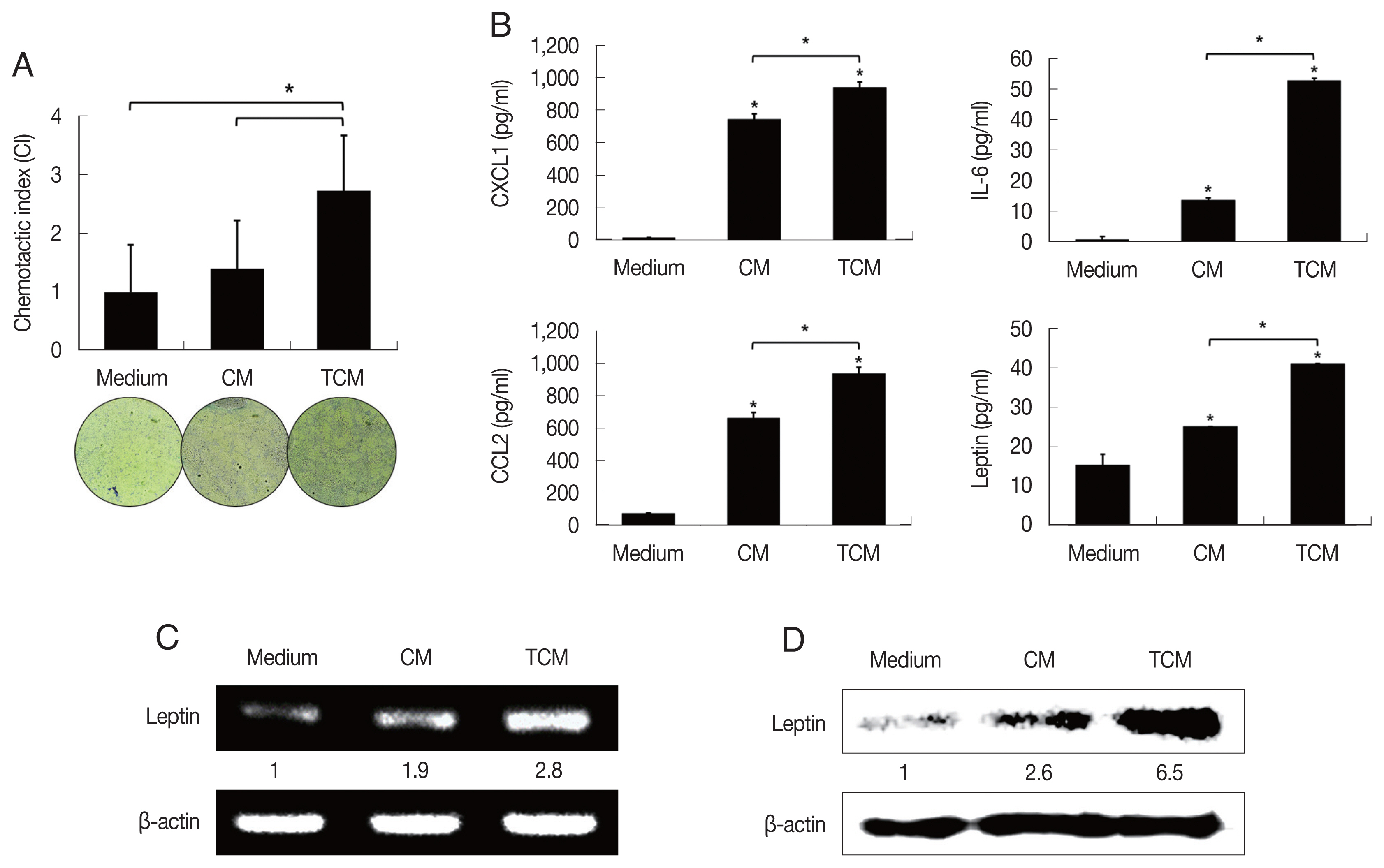
Migration of preadipocytes, and adipokine expression in adipocytes exposed to TCM. The effect of TCM on the migration of preadipocyte was examined. The migration of 3T3-L1 cells was assessed by counting the number of cells that migrated through a membrane to a lower well containing TCM or CM over 24 hr. The graph shows relative preadipocyte migration (A). Production of adipokines by mature adipocytes exposed to TCM was measured by ELISA (B). mRNA and protein levels of leptin produced by mature adipocytes exposed to TCM were detected by RT-PCR (C) and western blotting (D), respectively. TCM, culture supernatant from BPH epithelial cells stimulated with T. vaginalis; CM, culture supernatant from BPH epithelial cells; Medium, DMEM medium. *P<0.05.
To determine whether the conditioned medium from T. vaginalis-stimulated BPH-1 cells (TCM) is involved in the production of leptin by adipocyte, 3T3-L1 preadipocytes were allowed to develop into mature adipocytes (Fig. 1C). The mature 3T3-L1 cells were then stimulated with TCM and found to release higher levels of CXCL1, IL-6, CCL2 than cells incubated with CM or medium only (P<0.05; Fig. 2B); especially, production of leptin mRNA and protein was also increased by TCM (Fig. 2C, D). This means that the T. vaginalis-induced inflammatory mediators of BPH-1 cells triggered the inflammatory response of adipocytes, including leptin production. The conditioned media from mature adipocytes incubated with TCM and CM were designated ATCM and ACM, respectively.
Expression of leptin receptor in prostate cells exposed to ATCM
The effects of leptin are mediated by the transmembrane leptin receptor (OBR), which belongs to the cytokine receptor superfamily [30]. It was also reported that OBR is expressed in BPH tissue [31]. To measure ATCM-induced expression of leptin receptor, WPMY-1 and BPH-1 cells were incubated in 10% ATCM, and OBR was detected by immunofluorescence microscopy and western blot assay. OBR levels were elevated in both sets of ATCM-treated cells although their levels were slightly lower than in cells exposed to recombinant leptin (Fig. 3).
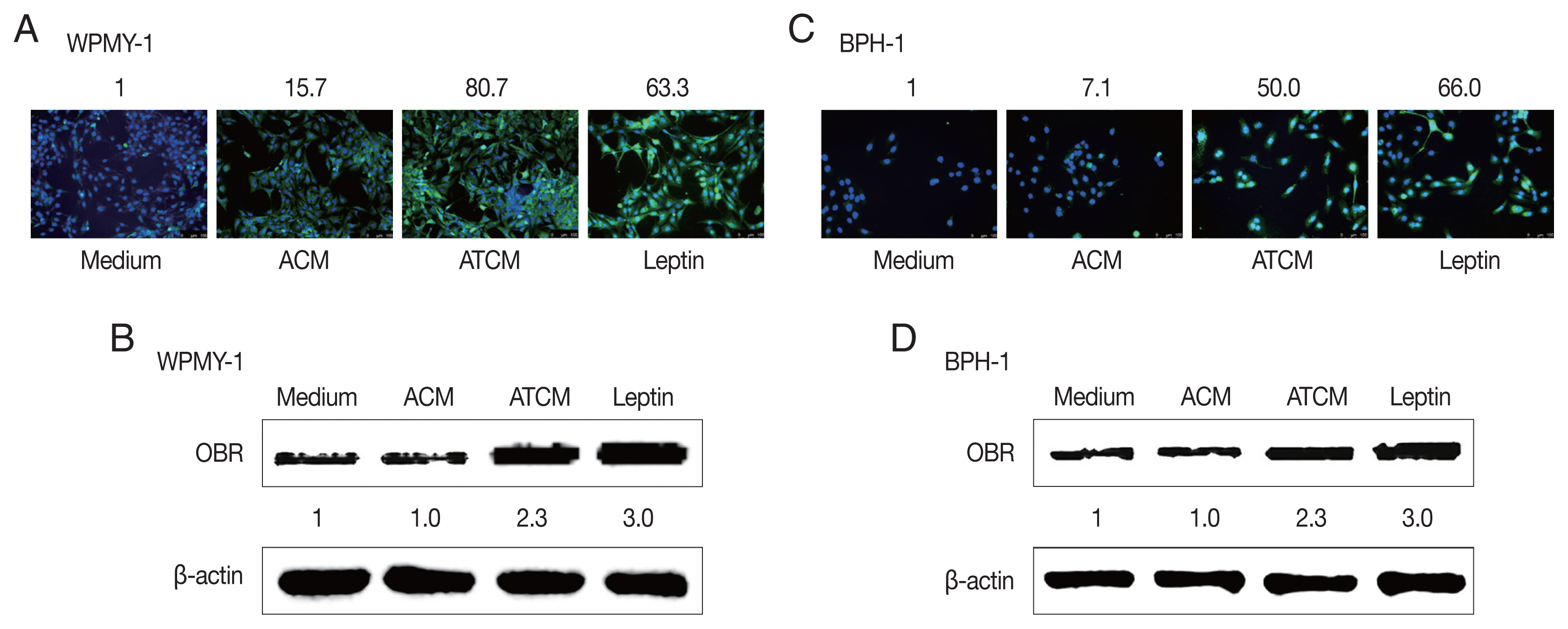
Increased expression of the leptin receptor in prostate cells treated with ATCM. Leptin receptor (OBR) expression in prostate cells treated with ATCM was observed by immunofluorescence and western blot assays. Expression of OBR increased in prostate stromal cells (A, B) and BPH epithelial (C, D) cells exposed to ATCM. ATCM, culture supernatant of adipocytes exposed to TCM; ACM, culture supernatant of adipocytes exposed to CM; TCM, culture supernatant of BPH epithelial cells exposed to T. vaginalis; CM, culture supernatant of BPH epithelial cells; Leptin, recombinant leptin; Medium, culture medium of prostate cells.
Proliferation of prostate cells is induced by ATCM
It is not clear whether leptin could influence on the growth of BPH although leptin was associated with proliferation and invasion of prostate cancer [32]. To determine whether conditioned media including leptin are involved in proliferation of prostate cells, BPH-1 and WPMY-1 cells were incubated with CM, TCM, ACM, and ATCM, and the proliferation was measured by BrdU and wound healing assay. The ATCM containing higher amount of leptin than other conditioned media increased wound healing (P<0.05; Fig. 4A, D) and number of BrdU positive cells (Fig. 4B, E) in both prostate cells. Our data suggest that ATCM stimulates the proliferation of prostate stromal and BPH epithelial cells. Interestingly, WPMY-1 cells treated with ATCM were observed to have slightly increased proliferation compared to the results of BPH-1 cells. In addition, although no invasiveness was observed in BPH-1 cells, WPMY-1 cells showed increased invasiveness (Fig. 4C). In BPH tissue, proliferation is known to be observed 9 times in epithelial cells and 37 times in stromal cells compared to normal prostate, and the overgrowth of the stromal cells is recognized as a characteristic of BPH pathogenesis [33]. In this experiment, the increased mobility, multiplication and invasiveness of the prostate stromal cell were in line with the characteristics of BPH tissue.
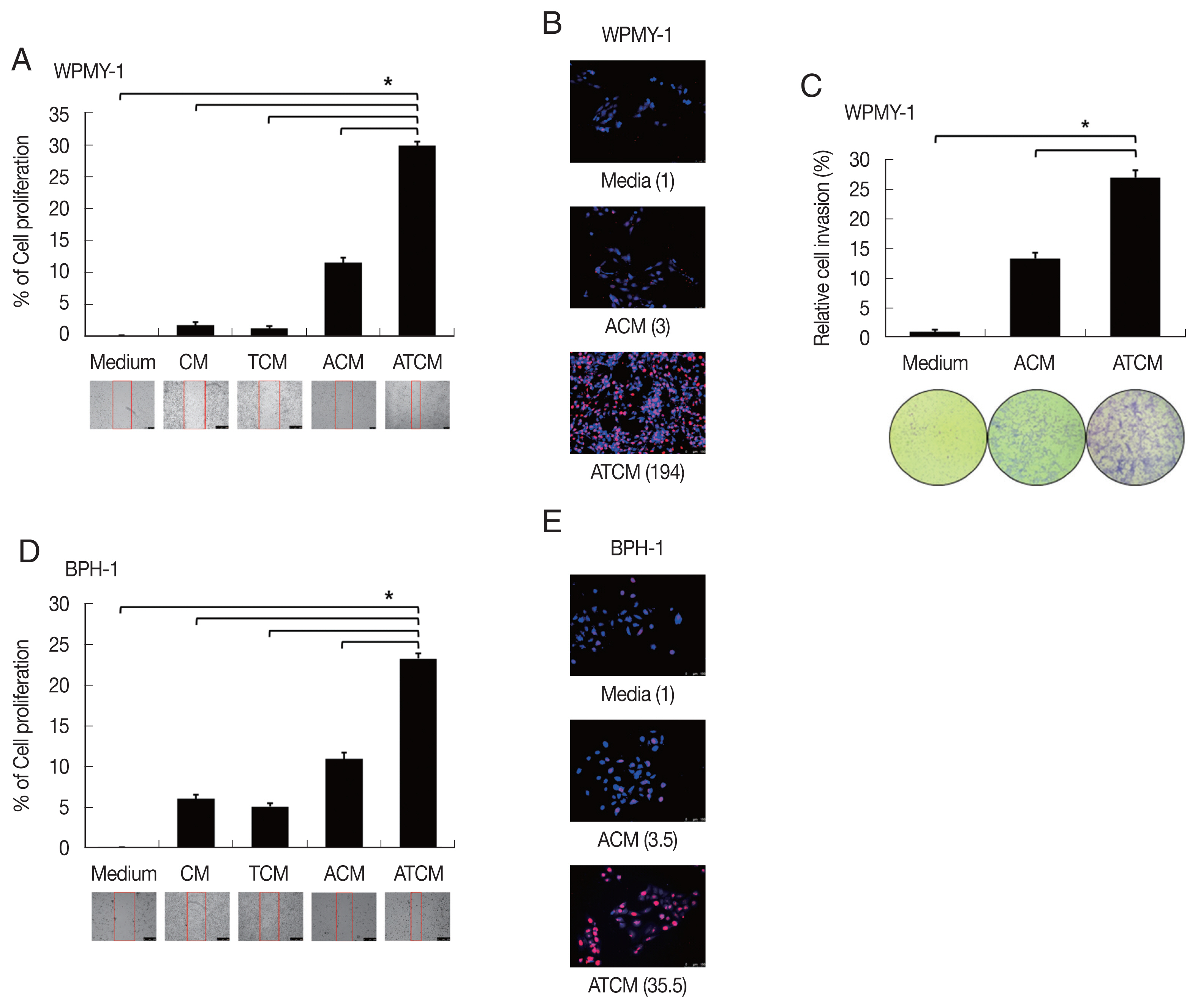
Increased proliferation of prostate cells incubated with ATCM. Wound healing and BrdU assays were used to measure the proliferation of prostate stromal cells (WPMY-1) and BPH epithelial cells (BPH-1) incubated with ATCM. Wound healing was observed with an inverted microscope (A, D) and DNA synthesis in proliferating cells was assessed with the bromodeoxyuridine (BrdU) assay. BrdU-positive cells were observed by fluorescence microscopy (B, E). To investigate the effect of ATCM on invasiveness, the matrigel invasion assay was used. WPMY-1 cells were more invasive towards ATCM than towards medium or ACM (C). ATCM, culture supernatant of adipocytes incubated with TCM; ACM, culture supernatant of adipocytes incubated with CM; TCM, culture supernatant of BPH epithelial cells incubated with T. vaginalis; CM, culture supernatant of BPH epithelial cells; Medium, culture medium used for all prostate cells. *P<0.05 versus ATCM.
Expression of leptin signaling molecules in prostate cells exposed to ATCM
Leptin binding to OBR has been reported to activate the classical JAK2/STAT3 pathway [30]. The JAK2-STAT3 pathway stimulates proliferation, angiogenesis, and anti-apoptotic events in normal and malignant cells expressing OBR. Leptin signaling also interacts with Notch, which is involved in tumor progression [23,34]. To probe whether the leptin signaling molecules are induced by ATCM, protein levels of leptin signaling molecules were measured in the prostate cells incubated with ATCM. Downstream leptin signaling components such as JAK2/STAT3, Notch1/Jagged1, and survivin also increased in response to ATCM in both sets of prostate cells (prostate stromal and BPH-1 cells) (Fig. 5). Similar results were obtained when recombinant leptin was used.
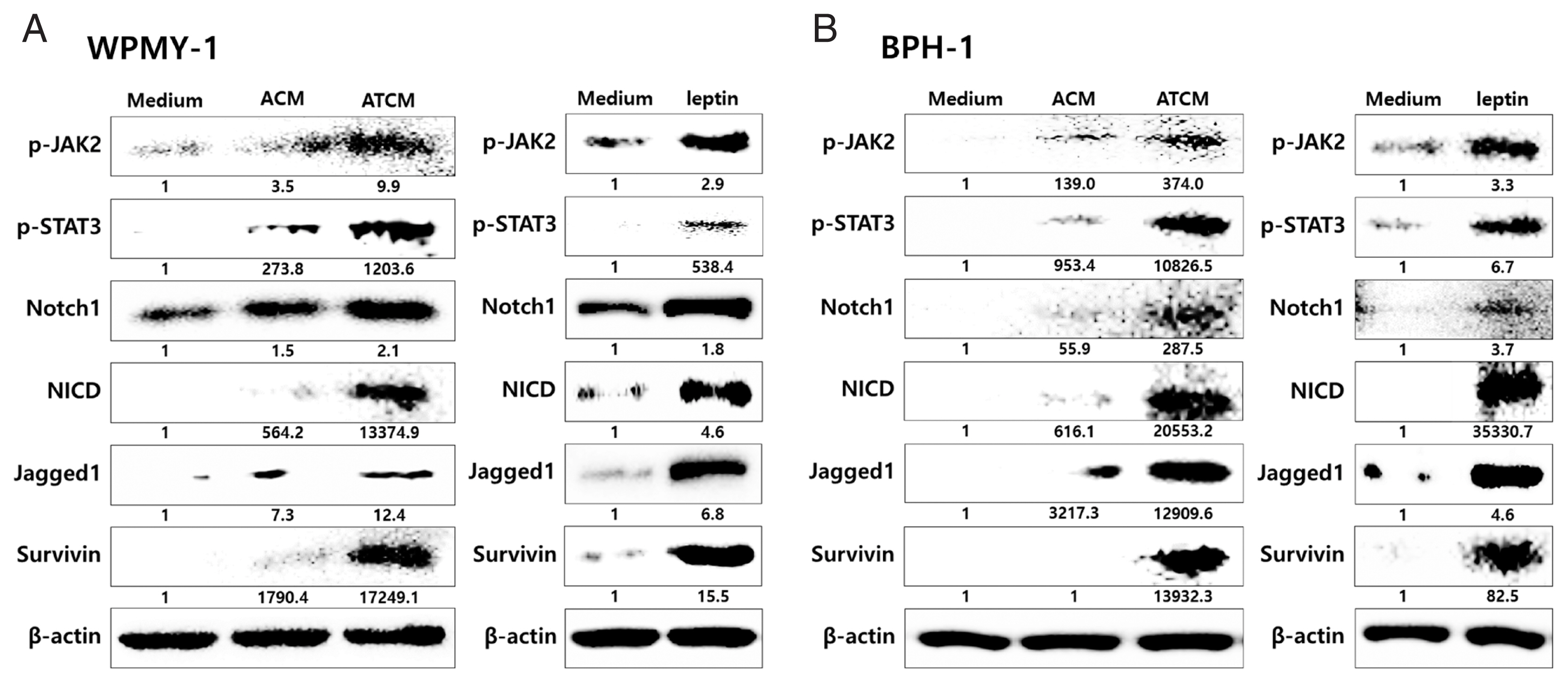
Increased expression of leptin signaling molecules in prostate cells exposed to ATCM. Expression of leptin signaling molecules such as JAK2/STAT3, Notch1/Jagged1, NICD and survivin was increased by treatment with ATCM or recombinant leptin in prostate stromal (A) and BPH-1 cells (B). ATCM, culture supernatant of adipocytes exposed to TCM; ACM, culture supernatant of adipocytes exposed to CM; TCM, culture supernatant of BPH epithelial cells exposed to T. vaginalis; CM, culture supernatant of BPH epithelial cells; Leptin, recombinant leptin; Medium, culture medium of prostate cells; NICD, Notch intracellular domain.
Inhibition of OBR reduces expression of leptin signal molecules and proliferation of Prostate cells
To investigate the role of OBR in leptin signaling of prostate cells exposed to ATCM, the cells were treated with ATCM together with anti-OBR antibody. Production of JAK2/STAT3 and Notch/Jagged was markedly inhibited by the anti-OBR antibody (P<0.05; Fig. 6). This indicates that ATCM activates the leptin signal pathway via OBR. When the OBR was blocked, ATCM-induced proliferation of prostate cells also decreased. Moreover, inhibition of leptin downstream signaling using a JAK inhibitor (ruxolitinib) or a Notch inhibitor (DAPT) reduced prostate cell proliferation (P<0.05; Fig. 7), which shows that the leptin signaling pathway contributes to proliferation. The use of isotype-matched antibodies as a control had no effect on cell proliferation (data not shown). Our findings indicate that leptin-OBR signaling is involved in the proliferation of WPMY-1 and BPH-1 cells.
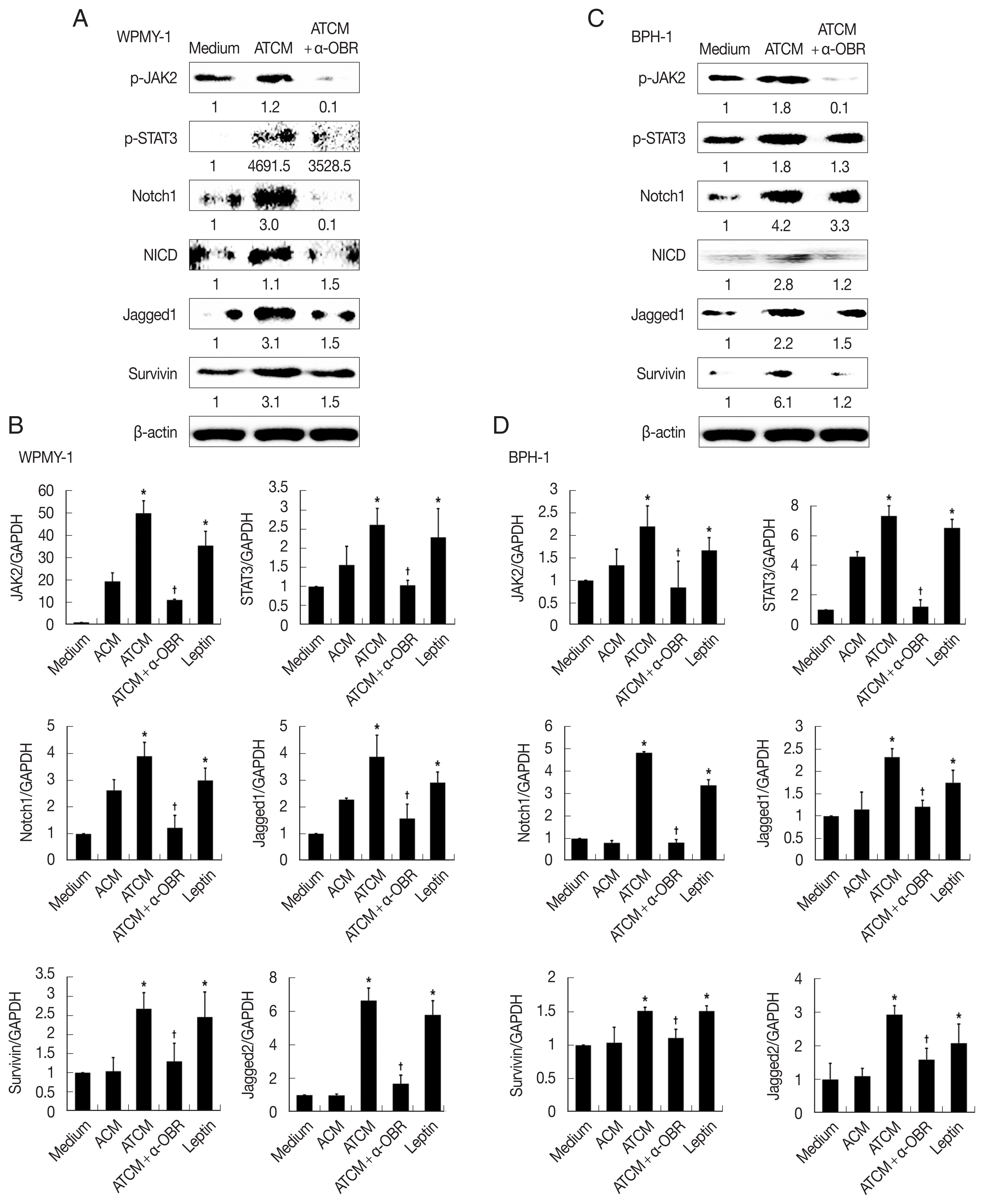
Blockade of the leptin receptor reduces the expression of downstream signaling molecules in prostate cells stimulated with ATCM. To investigate the expression of downstream leptin signaling molecules in prostate cells exposed to ATCM, prostate stromal cells (WPMY-1) and BPH epithelial cells (BPH-1) were incubated with ATCM or ATCM+anti-OBR antibody (α-OBR). Protein and mRNA levels were measured by western blot (A, C) and Q-PCR (B, D), respectively. ATCM, culture supernatant of adipocytes incubated with TCM; ACM, culture supernatant of adipocytes incubated with CM; TCM, culture supernatant of BPH epithelial cells incubated with T. vaginalis; Medium, culture medium of prostate cells; NICD, Notch intracellular domain. *P<0.05 versus medium. †P<0.05 versus ATCM.
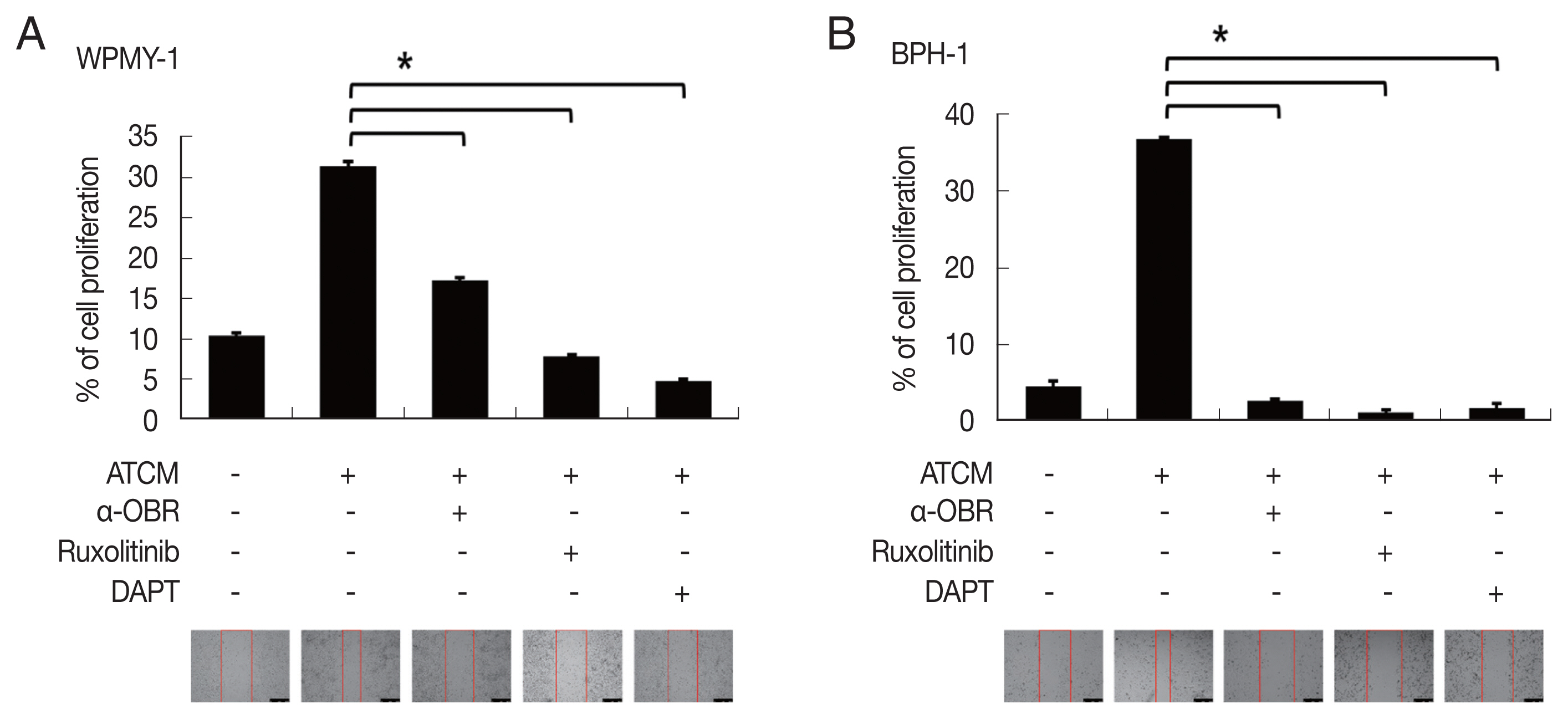
Inhibition of leptin signaling decreases the proliferation of prostate cells. To confirm the involvement of leptin signaling in the growth of prostate stromal (WPMY-1) and BPH epithelial (BPH-1) cells, the leptin receptor (OBR), JAK or Notch were inhibited, and the wound healing assay was conducted. Anti-OBR antibody (α-OBR) and the JAK inhibitor (ruxolitinib) and Notch inhibitor (DAPT) decreased the proliferation of both prostate cell types in response to ATCM. ATCM, culture supernatant of adipocytes incubated with TCM; TCM, culture supernatant of BPH epithelial cells incubated with T. vaginalis. *P<0.05 versus ATCM.
OBR expression slightly increased in BPH tissue in obese BPH patients compared to lean patients
A total of 94 patients with benign prostatic hyperplasia who underwent surgical resection in Hanyang University Hospital (Seoul, Korea) from 2016 to 2018 were identified. Among them, ten obese and 10 lean patients were categorized by BMI level. The patients whose mean of BMI level was 28 classified into the obese group. The lean group has had an average BMI level of 23. OBR expression was detected immunohistochemically with rabbit polyclonal anti-OBR antibody. Intensity of OBR expression was represented as a numerical score from 0 to 5 based on proportion of clusters of immunopositive cells. The OBR was slightly more expressed in human BPH tissue from obese patients than lean patients (Fig. 8).
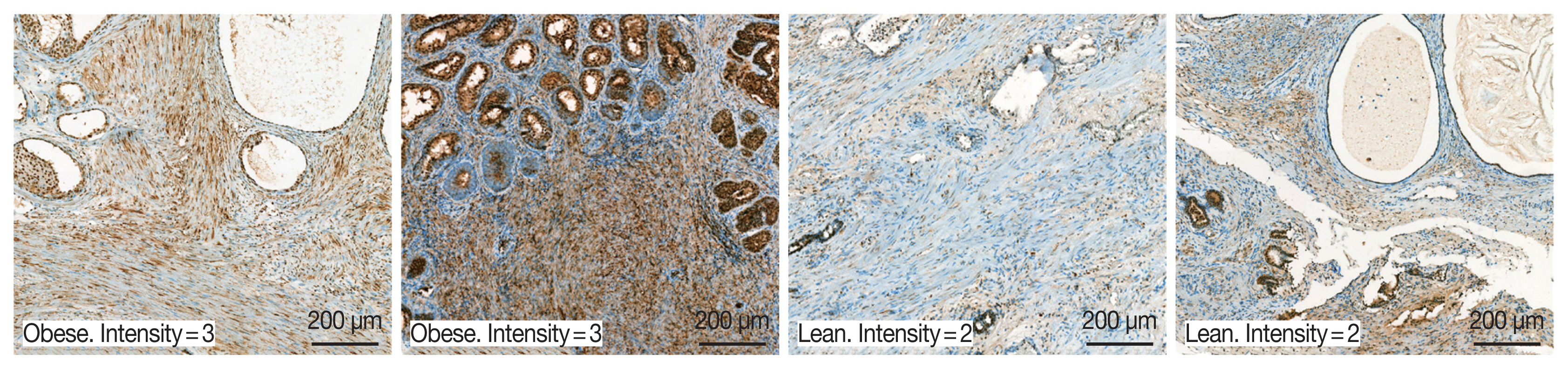
Leptin receptor (OBR) expression slightly increased in BPH tissue in obese BPH patients compared to lean patients. OBR expression was detected immunohistochemically with rabbit polyclonal anti-OBR antibody. Intensity of OBR expression was represented as a numerical score from 0 to 5 based on proportion of clusters of immunopositive cells. The OBR was slightly more expressed in human BPH tissue from obese patients than lean patients. Bar=200 μm.
DISCUSSION
Trichomonas vaginalis is mostly known as a surface-dwelling parasite, but it has been detected in endo-epithelial cells and in sub-epithelial tissues because of its ability to invade [5,6]. Because T. vaginalis infection has been found in over 70% of the male sexual partners of infected women, the number of infected men must be not ignored [35]. 19.9% of male nongonococcal urethritis (NGU) patients were reported to be infected with T. vaginalis, and BPH may be associated with chronic retention of T. vaginalis in the prostate gland [5,36]. Microenvironmental factors such as infection and wounding may alter cellular equilibria leading to BPH or prostate cancer [37]. A little is known about the proliferative mechanism of prostate cells. Siejka et al. [38] reported that bidirectional stromal-epithelial interactions occur in the prostate gland; when supernatants of prostate stromal cells (or BPH epithelial cells) were added to BPH epithelial cells (or prostate stromal cells), proliferation increased. In this context, our previous studies showed that the proliferation of inflamed BPH epithelial cells infected with T. vaginalis induced the multiplication of prostate stromal cells via crosstalk with mast cells, and IL-6 released by the proliferating prostate stromal cells stimulated the proliferation of BPH epithelial cells [26,27].
As people’s lifestyles have changed, obesity has become an important issue even in Asia. Obesity is related to social problems as well as personal health care. It causes many diseases, and is closely linked to diabetes, hypertension, malignancy, etc. [39]. Adipose tissue affects the endocrine and immune systems by secreting cytokines and hormones. These cytokine-like mediators produced by adipose tissue are called adipokines, and include leptin, adiponectin and resistin [40]. Leptin was first identified as a key molecule in the regulation of food intake and body weight. It also has a role in wound healing, reproduction, hematogenesis, and regulation of insulin and testosterone [41,42].
Clinical BPH, a condition involving abnormal progressive growth of prostate tissue is a common disease in elderly men, and causes lower urinary tract syndrome [43]. As the average human lifespan increases, it is becoming recognized as an important disease that affects quality of life and in which BPH, hormonal alterations, metabolic syndrome and obesity play key roles [13–15]. Obesity, central or general, is thought to be a risk factor for BPH [17]. The molecular mechanisms associated with the progression and aggravation of BPH in obesity are not known. In this study, we investigated whether BPH proliferation was induced by adipocyte leptin, which was stimulated by T. vaginalis infection in prostate cells.
The role of leptin has been much studied in breast cancer; Leptin may act via JAK/STAT signaling to increase breast cancer tissue proliferation [44]. It has been implicated in the higher incidence of aggressiveness, and appears to be involved in the proliferation and migration of breast cancer cells [45]. Guo et al. [46] have been reported that leptin induces Notch, and the Notch target gene, IL-1, and VEGF, and their levels of these molecules in breast cancers are correlated with the occurrence of metastases and lower survival. However, the function of leptin in the prostate has not been much studied. There are a few reports that leptin is associated with proliferation of the prostate; Leptin seems to have an important role in maintaining the physiological growth of the prostate. In hyperplasic prostate tissue, leptin treatment increased cellular proliferation and decreased apoptosis [47]. It is also reported to stimulate the proliferation of a BPH-1 cell and a prostate cancer cell line (PC-3 cell) via estrogen metabolism [48]. Leptin expression in prostate cancer tissue is significantly higher than in BPH, although expression of the leptin receptor (OBR) was similar in BPH and prostate cancer tissue [31]. Therefore, it was recently suggested that leptin might be a novel target for therapeutic intervention in prostatic disorders [48]. In agreement with the above reports, proliferation of prostate cells in our study was upregulated upon exposure to adipocyte-conditioned medium (ATCM) which contained leptin, and inhibition of the OBR reduced leptin-induced proliferation. Thus, this study provides the first experimental evidence that the leptin-OBR signal pathway plays a role in prostate cell proliferation.
There are 6 isoforms of OBR, which exerts various physiological effects in response to binding by leptin, which is a 16 kDa non-glycosylated protein encoded by the Ob gene [49]. OBR belongs to a superfamily of class I cytokine receptors, which typically contain a cytokine receptor-homologous domain in their extracellular regions [19]. As with other class I cytokine receptors, the leptin signal is reported to be transmitted mainly by the JAK/STAT pathway. Binding of leptin to OBR leads to tyrosine phosphorylation of JAK1 and JAK2, and phosphorylation of downstream STAT transcription factors [50]. In the case of lung cancer cell, leptin was shown to promote growth, invasiveness, and migration with concomitant activation of JAK/STAT, PI3K/AKT and ERK signaling [22]. In this study, adipocyte leptin was found to stimulate the OBR and the JAK2/STAT3 signal pathway in prostate cells; inhibition of the OBR and JAK decreased leptin-induced proliferation of prostate cells. Besides, the OBR was slightly more expressed in BPH tissue in obese than in lean patients (Fig. 8).
Notch is a downstream signal of leptin, and is highly conserved in evolution. The Notch signal is very important in determining cell-fate decisions from proliferation, differentiation and apoptosis to cancer cell invasion and metastasis [51]. The Notch family consists of 4 receptors (Notch 1–4) and has 5 ligands named Jagged 1, 2, Delta-like 1, 3, and 4 [22]. In response to ligand binding, the Notch receptor undergoes proteolytic cleavage, resulting in the release of the Notch intracellular domain (NICD). NICD then enters the nucleus and forms a complex with RBP-jκ (also known as CSL) and histone acetyltransferase (CBP/p300) leading to the expression of Notch target genes such as survivin [52]. The notch signal has been reported to play critical roles in intraepithelial neoplasia formation in prostate cancer, and in proliferation and angiogenesis in breast and pancreatic cancers [53,54]. But the Notch signal hitherto had no known role in BPH enlargement. In this study, the leptin-OBR signal activated Notch signaling, and inhibition of Notch reduced the proliferation of prostate stromal cells and BPH epithelial cells. This result suggests for the first time that Notch signaling may be involved in the proliferation of BPH cells via the leptin-OBR signal pathway. Meanwhile, Mullen et al. [23] proposed a signal cascade that leptin induces Notch in breast cancer through JAK2/STAT3. Although our study revealed that JAK2/STAT3 is involved in the leptin-OBR signaling pathway, the signaling cascade has not been well documented. Further research is needed.
Survivin, a member of the inhibitor-of-apoptosis protein family, mainly acts to suppress apoptosis and regulate cell division [55]. Although not expressed in normal adult differentiated tissue, it is present in most cancers, regardless of lineage, differentiation and histological type [56]. Survivin expression correlates with unfavorable disease outcomes through increased aggressiveness and metastasis in colorectal, pancreatic, breast and ovarian cancers [55,57,58]. Downstream effector molecules of leptin signaling (such as Stat3 and Akt) are reported to participate in the regulation of survivin expression [59]. In our case, expression of survivin increased in response to ATCM. Inhibition of the leptin-OBR pathway reduced survivin levels and consequently decreased leptin-induced proliferation of the prostate cells. This result is probably the first evidence that survivin is involved in the leptin-induced proliferation of prostate cells.
In summary, inflammatory mediators released by BPH epithelial cells in response to infection with T. vaginalis induce migration and activation of adipocytes. The activated adipocytes then stimulate the proliferation of prostate cells through leptin-OBR signaling. Therefore, it is likely that T. vaginalis contributes to prostate enlargement via adipocyte leptin released as a result of inflammation of the prostate (Fig. 9).
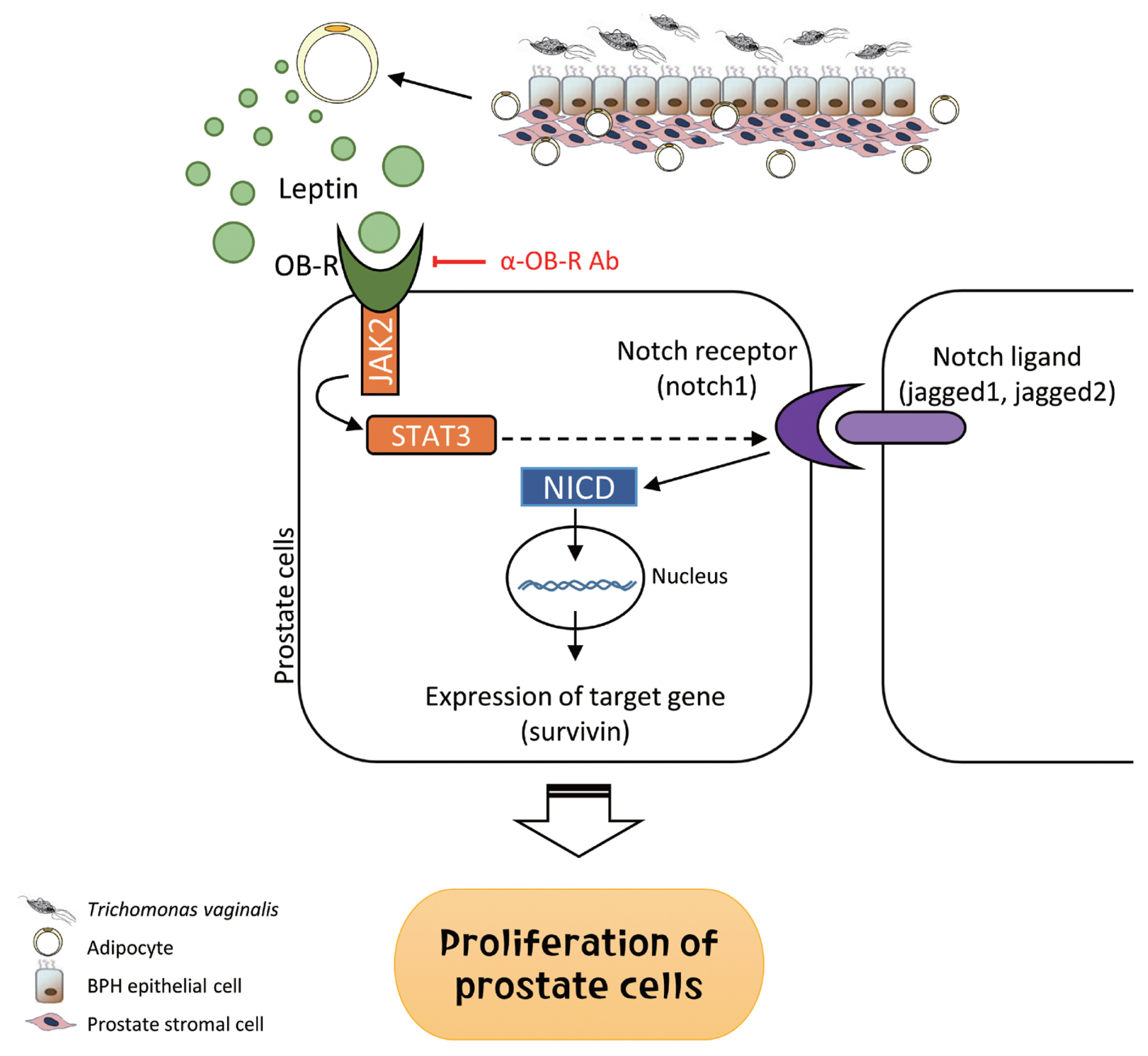
Role of adipocyte leptin in the proliferation of prostate cells infected with Trichomonas vaginalis. We investigated whether inflammation from Trichomonas vaginalis infection is involved in the proliferation of benign prostatic hyperplasia (BPH) in the obese state. Inflammatory mediators released by BPH epithelial cells infected with T. vaginalis triggered adipocyte migration and activation. Leptin produced from the activated adipocytes induced prostate cell proliferation through the leptin-OBR signaling pathway.
ACKNOWLEDGMENT
This research was supported by the Basic Science Program through the National Research Foundation of Korea (NRF) funded by the Ministry of Science and ICT (NRF-2017R1A2B4002072).
Notes
The authors declare that they have no conflicts of interest.