Characterization of a Peptide Antibody Specific to the Adenylyl Cyclase-Associated Protein of Acanthamoeba castellanii
Article information
Abstract
Acanthamoeba keratitis (AK) is a rare infectious disease and accurate diagnosis has remained arduous as clinical manifestations of AK were similar to keratitis of viral, bacterial, or fungal origins. In this study, we described the production of a polyclonal peptide antibody against the adenylyl cyclase-associated protein (ACAP) of A. castellanii, and evaluated its differential diagnostic potential. Enzyme-linked immunosorbent assay revealed high titers of A. castellanii-specific IgG and IgA antibodies being present in low dilutions of immunized rabbit serum. Western blot analysis revealed that the ACAP antibody specifically interacted with A. castellanii, while not interacting with human corneal epithelial (HCE) cells and other causes of keratitis such as Fusarium solani, Pseudomonas aeruginosa, and Staphylococcus aureus. Immunocytochemistry (ICC) results confirmed the specific detection of trophozoites and cysts of A. castellanii co-cultured with HCE cells. The ACAP antibody also specifically interacted with the trophozoites and cysts of 5 other Acanthamoeba species. These results indicate that the ACAP antibody of A. castellanii can specifically detect multiple AK-causing members belonging to the genus Acanthamoeba and may be useful for differentially diagnosing Acanthamoeba infections.
INTRODUCTION
Acanthamoeba keratitis (AK) is a rare infectious disease affecting the eye that can lead to permanent vision loss if not treated properly [1]. To prevent such disastrous consequences from occurring, a rapid and accurate AK diagnosis is crucial. In AK patients, bacterial or fungal coinfections are common. Members of the genera Staphylococcus and Fusarium were reported as the most common pathogens of bacterial and fungal origins encountered by AK patients, respectively [2,3]. Since symptoms of AK can be quite similar to those of bacterial and fungal keratitises, it is often misdiagnosed [4]. Therefore, the possibility of these pathogens coinhabiting the cornea along with Acanthamoeba spp. should always be considered to deliver an accurate diagnosis and appropriate treatments.
Currently, most cases of AK have been diagnosed by confocal microscopy, corneal scrapings, Acanthamoeba culture on agar plates, polymerase chain reaction amplification, and histopathological examinations [5,6]. Immunoassays using specific antibodies are also important techniques for the detection of Acanthamoeba. Four monoclonal antibodies (AMEC1, AMEC3, MTAC1, and MTAC3) generated by mixtures of A. castellanii trophozoites and cysts, a monoclonal antibody against a CPA2 transporter of A. castellanii, and a monoclonal antibody against a mannose-binding protein of A. culbertsoni have been produced to support AK diagnosis [7–9]. Polyclonal antibodies against inosine-uridine preferring nucleoside hydrolase (IPNH) and chorismate mutase (CM) of A. castellanii have also been produced for specific detection of Acanthamoeba [10,11]. Despite the ongoing development of diagnostic techniques, the methods are not yet widely used. Therefore, sensitive and rapid differential diagnostics are still needed to effectively diagnose and treat AK.
Adenylyl cyclase-associated protein (ACAP) was first identified in Saccharomyces cerevisiae, and CAP (adenylyl cyclase associated protein) homolog was isolated from Dictyostelium discoideum [12,13]. Some of the major functions of this protein involve remodeling of the actin cytoskeleton and regulating cell motility [14]. CAP homologs exist in all species, including bacteria, yeasts, fungi, plants, and mammals [15]. Many studies have shown that CAPs are involved in various cellular and pathological processes by regulating the actin cytoskeleton and the Ras/cAMP pathway [16]. In mammals, 2 CAP isoforms have been identified. CAP1 is expressed in almost all tissues and cells, whereas CAP2 has specific expressions in skeletal and cardiac muscles, brains, and skin [14]. CAP2 is a biomarker with diagnostic and prognostic values in human gastric and breast cancer [17,18]. CAP2 is also a potential diagnostic biomarker for early hepatocellular carcinoma [19]. In recent years, many studies have been conducted on CAP as a biomarker for the diagnosis of human diseases. However, studies reporting the application of CAP to detect parasitic organisms are extremely limited.
In our previous study, we found that the gene expression of ACAP of A. castellanii is upregulated to a greater extent in the pathogenic strain than in the non-pathogenic strain [20]. Based on this observation, we hypothesized that ACAP could be used to specifically detect pathogenic strains of Acanthamoeba. Thus, we produced a polyclonal peptide antibody targeting the ACAP of pathogenic A. castellanii, and evaluated its diagnostic potential. The objective of this study was to describe the production of ACAP antibodies and their specificities to several pathogenic Acanthamoeba spp. using enzyme-linked immunosorbent assay (ELISA), western blot analysis, and immunocytochemistry (ICC) assay.
MATERIALS AND METHODS
Cell cultures
Human corneal epithelial (HCE) cells (PCS-700–010) and Acanthamoeba castellanii Castellani (ATCC 30868) were purchased from the American Type Culture Collection. A. castellanii Neff, A. hatchetii, A. polyphaga, A. culbertsoni, and A. royreba were kindly provided by Prof. Ho-Joon Shin at Ajou University (Suwon, Korea). Fusarium solani, Pseudomonas aeruginosa, and Staphylococcus aureus were obtained from the Korea Centers for Disease Control & Prevention (NCCP 32678, NCCP 16091, and NCCP 15920). HCE cells were cultured at 37°C with 5% CO2 in endothelial cell growth medium kits (KGM BulletKit) (Lonza, Portsmouth, New Hampshire, USA). Acanthamoeba trophozoites were cultured in Peptone-Yeast-Glucose (PYG) media at 25°C for 5 days, and cysts were induced in encystment media (95 mM NaCl, 5 mM KCl, 8 mM MgSO4, 0.4 mM CaCl2, 1 mM NaHCO3, and 20 mM Tris-HCl, pH 9.0) at 25°C. F. solani was cultured using Sabouraud Dextrose (SD) media at 25°C, and P. aeruginosa and S. aureus were cultured on Brain Heart Infusion (BHI) media at 37°C incubators.
Peptide antibody production of adenylyl cyclase-associated protein
The peptide sequence of adenylyl cyclase-associated protein used as the immunogen is as follows: NH2-QRELLYKASQSKKPDATTFG-C-COOH. Synthesized peptide and the antibody raised against the peptide were purchased from AbFRONTIER (AbFRONTIER, Seoul, Korea). Briefly, 2 NZW rabbits were injected intradermally with 1.0 mg of peptide-KLH conjugates with complete Freund’s adjuvant on days 0, and 0.5 mg of the peptide with incomplete Freund’s adjuvant on days 28, 42, and 56. The animals were bled on days 35 and 49. After the 3rd immunization, the antisera titer was assessed using an indirect ELISA with the peptide-BSA conjugates as coating antigens until the titer plateaued. After the last immunization, blood samples were acquired by cardiac puncture from each rabbit.
Enzyme-linked immunosorbent assay (ELISA)
Acanthamoeba-specific antibody responses against Acanthamoeba cell lysates (102 to 10−3 μg/μl) from serially diluted sera (1:5 to 1:50,000) were determined using ELISA as described previously [11]. Briefly, 96-well plates were coated with antigens in carbonate coating buffer overnight at 4°C. Plates were washed 3 times with PBST and blocked with 0.2% gelatin for 2 hr at 37°C. Sera diluted in PBS were inoculated into wells and incubated at 37°C for 1 hr. Horseradish peroxidase (HRP)-conjugated anti-rabbit IgG or IgA (Sigma-Aldrich, St. Louis, Missouri, USA) was added at 1:1,000 dilution in PBS and incubated at 37°C for 1 hr. O-phenylenediamine substrate (Zymed, San Francisco, California, USA) was dissolved in citrate-phosphate buffer with H2O2. The optical density values at 450 nm were read using EZ Read 400 microplate reader (Biochrom Ltd., Cambridge, UK). The sera of the unimmunized mice were used as a negative control.
Western blot
To verify the specificity of the ACAP antibody, western blotting was conducted using HCE cells, A. castellanii, F. solani, S. aureus, and P. aeruginosa [11]. Briefly, each cell lysates were confirmed on 10% SDS-PAGE and transferred to a nitrocellulose membrane. The membrane was blocked with 5% skim milk in TBST for 2 hr and incubated overnight at 4°C with the ACAP antibody (1:1,000). The membrane was washed with TBST and probed with HRP-conjugated anti-rabbit IgG (Sigma-Aldrich) (1:5,000) for 1 hr at RT. Bands were developed in the darkroom using Clarity Enhanced Chemiluminescence reagent (Thermo Fisher, Waltham, Massachusetts, USA) on x-ray films.
Immunocytochemistry (ICC)
To confirm the specificity of the ACAP antibody, an immunocytochemistry assay was conducted using HCE cells, Acanthamoeba spp. trophozoites, and cysts [11]. HCE cells (3×105 cells) were cultured on sterile cover glass in a 6-well plate. The following day, they were co-cultured with Acanthamoeba trophozoites (5×105 cells), and cysts (5×105 cells) for 5 hr at 37°C with 5% CO2. After washing with PBS, the cells were fixed with ice-cold 100% methanol for 5 min at RT. Cells were washed and permeabilized with PBST for 10 min at RT. The cells were washed and blocked using blocking buffer (1% bovine serum albumin and 22.52 mg/ml glycine in PBST) for 30 min at RT. The cells were incubated overnight at 4°C with ACAP antibody (1:200) in blocking buffer and probed with CruzFluorTM 555 (CFL 555)-conjugated anti-rabbit IgG (1: 400; Santa Cruz, Dallas, Texas, USA) for 1 hr at RT. After washing, cells were stained with VECTASHIELD mounting medium (Abcam, Burlingame, California, USA) and observed under a fluorescent microscope (Leica DMi8, Wetzlar, Germany).
Statistical analysis
Student’s t-test was performed using GraphPad Prism version 5 (San Diego, California, USA). Data are expressed as mean± SD. Statistical significance between the means of groups was denoted using an asterisk. P values less than 0.05 was considered statistically significant (*P<0.05, **P<0.01, and ***P< 0.001).
RESULTS
Sequence analysis of ACAP of A. castellanii for antigen preparation
The adenylyl cyclase-associated protein (ACAP) of A. castellanii consists of 1,458 bp and encodes 485 amino acids with a calculated mass of 53.35 kDa (GeneBank: MW683237.1). To design a peptide antigen with optimal antigenicity, amino acid sequences of ACAP of A. castellanii were compared with those of Homo sapiens (ACAP1 and ACAP2), F. oxysporum, S. aureus, and P. aeruginosa (Fig. 1). Based on the secondary structure analysis, hydrophobicity, and amino acid homology search, amino acids at positions 90–109 were selected for peptide antibody production (boxed area in Fig. 1). Amino acid sequence homology results showed that ACAP of A. castellanii showed 40.6%, 40.2%, 15.5%, 24.3%, and 19.2% similarity with those of H. sapiens (ACAP1 and ACAP2), F. oxysporum, S. aureus, and P. aeruginosa, each respectively (Table 1).
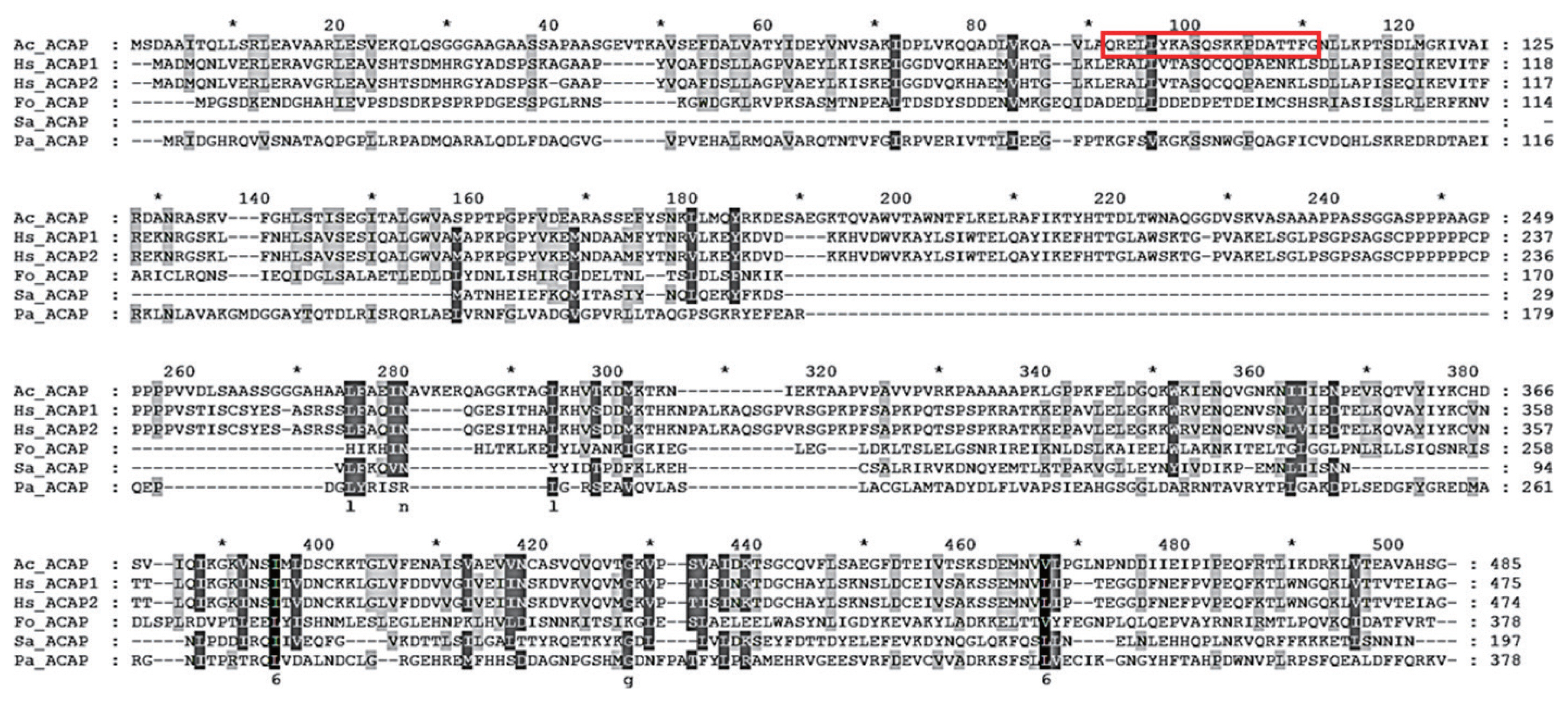
Multiple sequence alignment of ACAPs. Aligned amino acid sequences of ACAP from A. castellanii (Ac_ACAP), H. sapiens (Hs_ACAP1 and Hs_ACAP2), F. oxysporum (Fo_ACAP), S. aureus (Sa_ACAP), and P. aeruginosa (Pa_ACAP) were compared. The deduced amino acid sequences were aligned by using ClustalW, and the degree of conservation is represented by different shading. The boxed sequence was used to raise the anti-ACAP polyclonal antibody.
Antibody responses to ACAP antigen
ELISA was performed to titrate A. castellanii-specific IgG and IgA antibodies in low dilutions of immunized rabbit serum (Fig. 2). Acanthamoeba-specific IgG and IgA antibody responses could be observed until 1:5,000 and 1:50 dilutions of immune sera, respectively (Fig. 2A, B). To establish the sensitivity of the ACAP antibody, antibodies were reacted with the serially diluted cell lysates of A. castellanii. The detection ranges for the Acanthamoeba-specific IgG and IgA were as low as 10−2 μg/μl and 100 μg/μl of cell lysates, respectively (Fig. 2C, D). These results indicated that a high level of anti-Acanthamoeba IgG antibody and a low level of anti-Acanthamoeba IgA antibody responses could be elicited by the A. castellanii ACAP immune sera.
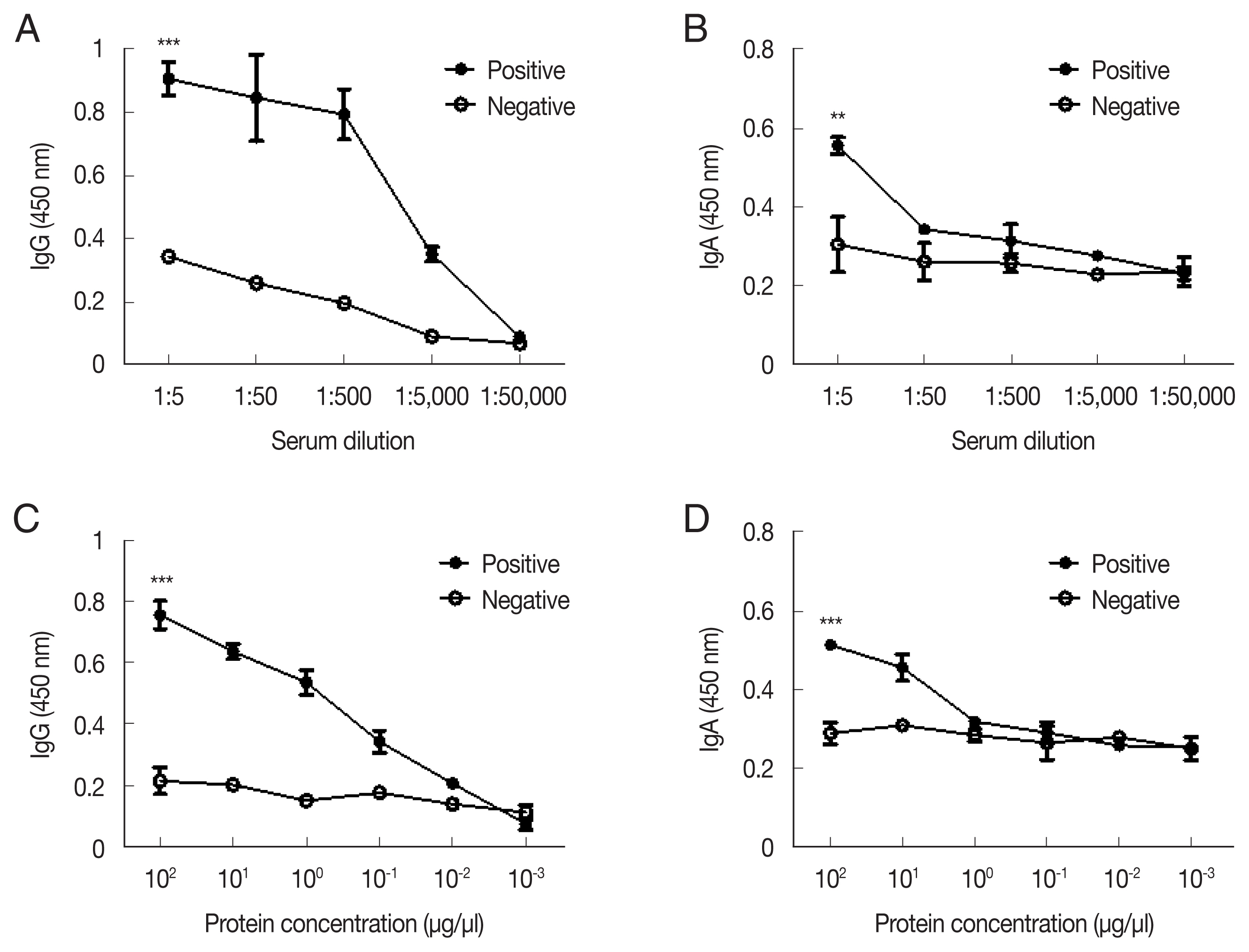
Antibody responses in rabbit antiserum to ACAP of A. castellanii. The ACAP-specific IgG and IgA antibody titers were measured by ELISA (A, B). Sera collected from the ACAP immunized rabbits were diluted at a ratio of 1:50,000. Sensitivities of these antibodies were assayed by serially diluted cell lysates of A. castellanii (C, D). Asterisks denote statistically significant differences between positive (●) and negative (○) serum (**P<0.01 and ***P<0.001).
Specificity of ACAP peptide polyclonal antibody
To investigate the specificity of the anti-ACAP peptide polyclonal antibody, western blot analysis was performed using cell lysates of HCE cells, A. castellanii, F. solani, S. aureus, and P. aeruginosa (Fig. 3). The ACAP antibody reacted with A. castellanii, while such strong interactions were not observed from HCE cells, F. solani, S. aureus, and P. aeruginosa. The estimated sizes of the ACAP of HCE cells, A. castellanii, F. oxysporum, S. aureus, and P. aeruginosa were 52.25kDa, 53.35 kDa, 41.58 kDa, 21.67 kDa, 41.58 kDa, each respectively (Table 1). Some faint bands appeared when F. solani, S. aureus, and P. aeruginosa lysates were probed with the ACAP antibody, but size differences indicate that they were of non-specific origin.
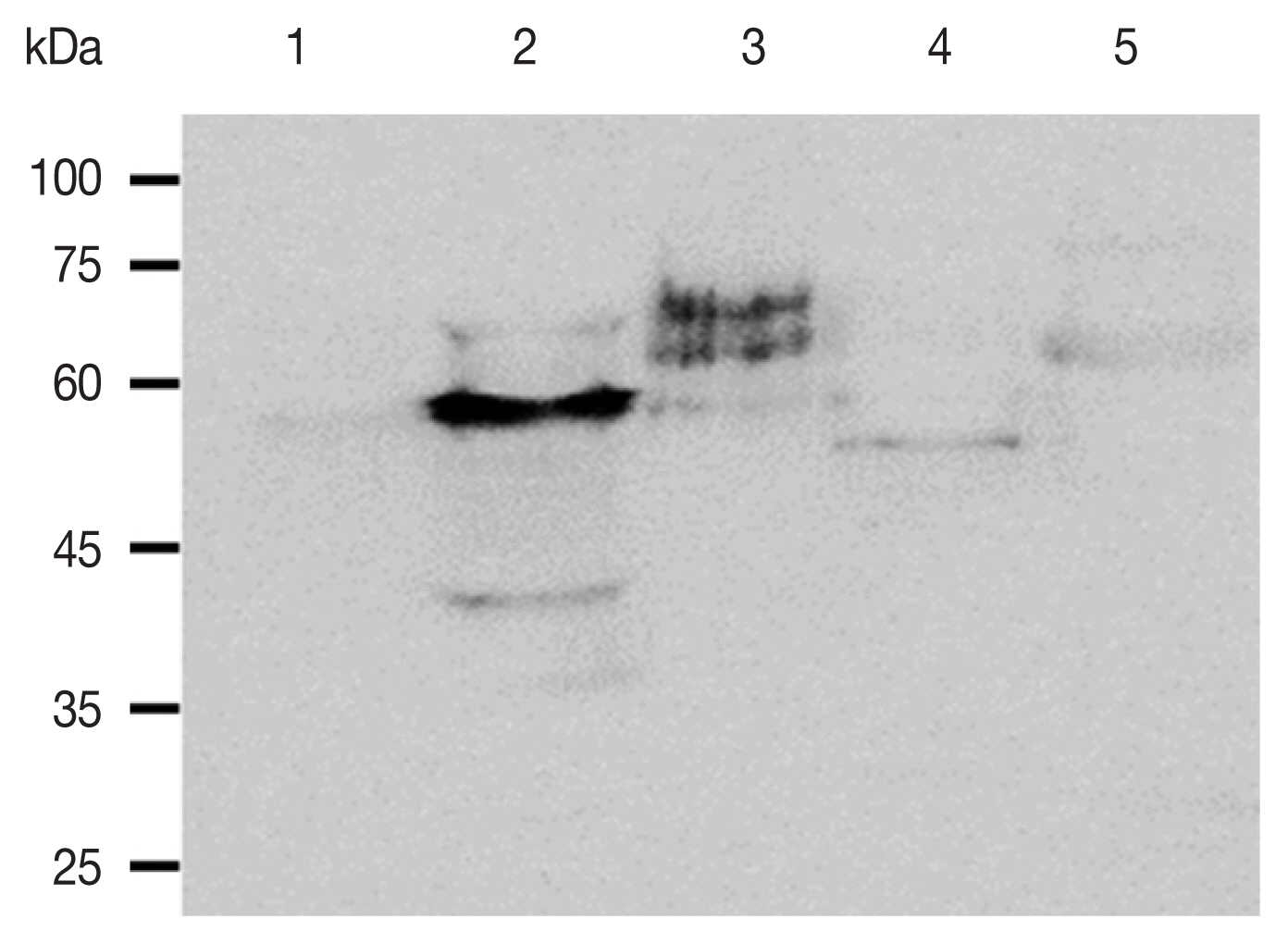
Specificity of the anti-ACAP antibody. Antibody specificity was determined by western blot analysis using cell lysates from different organisms. Lane 1; HCE cells, Lane 2; A. castellanii, Lane 3; F. solani, Lane 4; S. aureus, Lane 5; P. aeruginosa.
To confirm the specificity of ACAP antibody to A. castellanii trophozoites and cysts, immunocytochemistry was performed using HCE cells co-cultured with Acanthamoeba (Fig. 4). The nuclei of HCE cells were stained with DAPI (blue), but HCE cells remained unstained with ACAP antibody labeled with CFL fluorophore (red). However, A. castellanii trophozoites (top row) and cysts (bottom row) showed strong interaction with the ACAP antibody (red). DAPI-stained nuclei of Acanthamoeba were faintly visible because the size of Acanthamoeba nuclei is much smaller than that of HCE cells. This result demonstrated that the ACAP peptide antibody of A. castellanii specifically detected the trophozoites and cysts of A. castellanii.
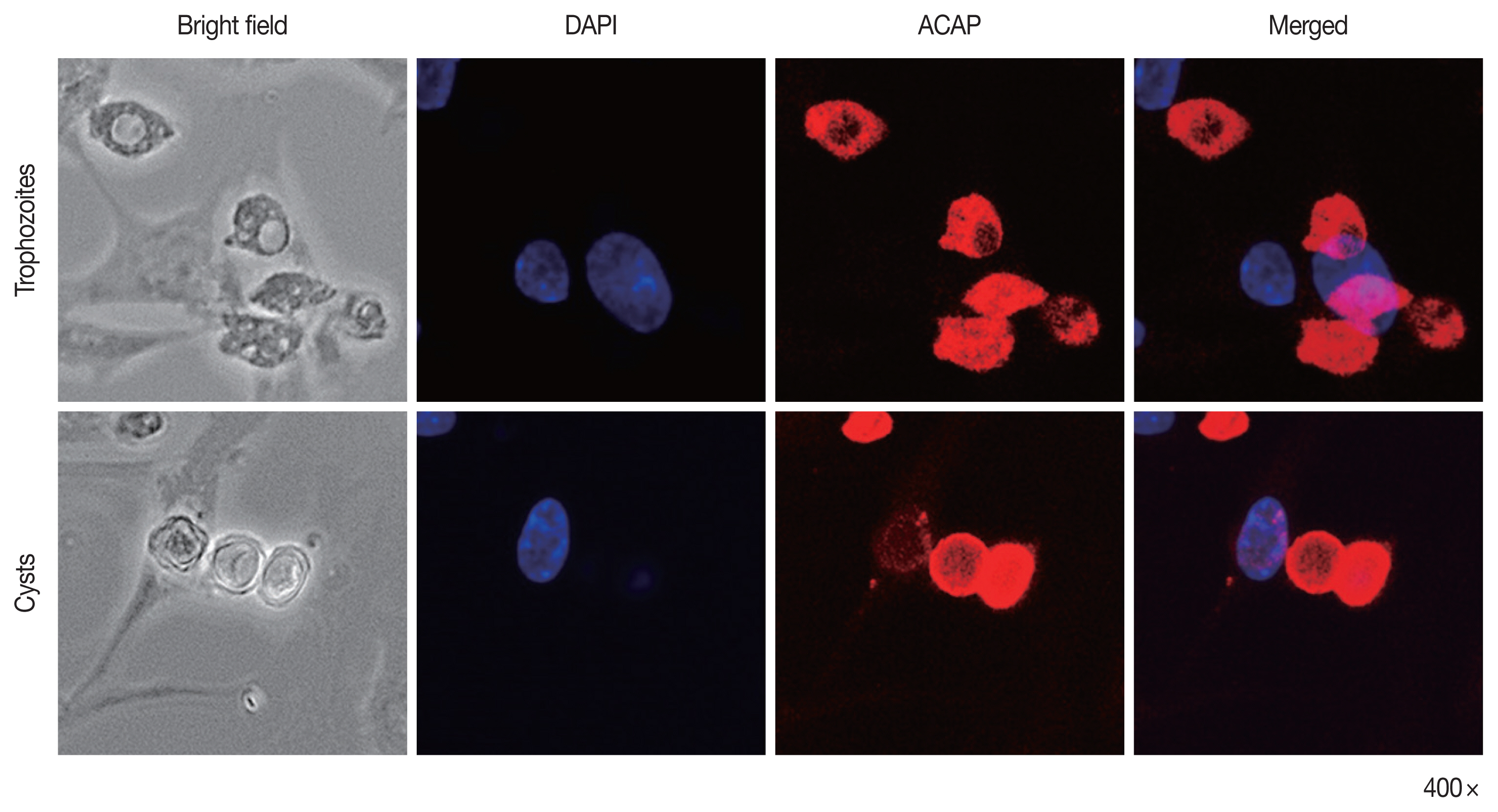
Immunocytochemistry assay of anti-ACAP antibody. HCE cells and A. castellanii trophozoites and cysts were co-cultured and observed under a fluorescent microscope. Bright-field, DAPI staining (blue), ACAP antibody combined with CFL-conjugated secondary antibody (red), and merged images were acquired.
The specificity of ACAP Antibody to other Acanthamoeba spp
For further verification of the specificity of the ACAP antibody, immunocytochemistry was performed using 5 different species of Acanthamoeba. (Table 2; Fig. 5). The trophozoites and cysts of A. castellanii Neff, A. hatchetti, A. polyphaga, A. culbertsoni, and A. royreba interacted with ACAP antibodies. However, the cysts of A. polyphaga did not specifically react with the ACAP antibody. This result showed that the ACAP antibody of A. castellanii was capable of detecting various species of Acanthamoeba trophozoites and cysts.
DISCUSSION
For effective treatment of AK, an accurate diagnosis in the early stages of infection is essential. Accordingly, sensitive and rapid differential diagnostic methods for AK have been studied. Currently, several studies investigating the diagnostic potential of antibody-based methods for AK have been reported [7–11]. In this study, we produced a polyclonal peptide antibody against the ACAP of A. castellanii and studied its specificity. This antibody was highly specific to 6 different species of Acanthamoeba.
Acanthamoeba exists either as an infective trophozoite form or a dormant cyst form. It is thought that trophozoite-derived proteases contribute to penetration and degradation of the stroma, and the severe pain associated with AK [21]. Encystation prolongs the infection and renders the Acanthamoeba highly resilient to therapeutic agents [22]. It has been reported that Acanthamoeba cysts are capable of surviving over 24 years [5]. Currently, the Gram stain and Giemsa stain are widely used for the rapid detection of Acanthamoeba cysts in corneal scrapings [23]. However, false-negative and false-positive results can occur if the Acanthamoeba spp. cannot be differentiated from surrounding tissue cells and other coinfecting pathogens [23]. We have previously reported the Acanthamoeba-specific antibodies targeting the IPNH and CM of A. castellanii [10,11]. Although these studies revealed that these antibodies could specifically identify trophozoites of Acanthamoeba spp. from other causes of keratitis, our previous studies did not investigate their diagnostic potential against cysts of Acanthamoeba spp. The ACAP participates in the activation of adenylyl cyclase, which is upregulated and induces prespore differentiation in Dictyostelium slugs [14,26]. It is presumed that the ACAP is also involved in the cyst formation of Acanthamoeba. The ACAP antibody described in this study strongly reacted with both trophozoites and cysts of the aforementioned 6 Acanthamoeba spp (Figs. 4, 5). Therefore, the presence of Acanthanthamoeba trophozoites and cysts could be easily confirmed by using this antibody.
Acanthamoeba spp. is distributed in natural environments. It has been reported that 50% to 100% of healthy people possess Acanthamoeba-specific antibodies [24,25]. However, AK patients have significantly higher anti-Acanthamoeba IgG antibody titers than healthy people [25]. In the present study, an immunized rabbit with ACAP of A. castellanii produced Acanthamoeba-specific high IgG but low IgA (Fig. 2). Acanthamoeba-specific IgG and IgA were demonstrated to be sensitive with a detection limit as low as 10 ng/μl and 1 μg/μl of Acanthamoeba cell lysate, respectively (Figs. 2C, D). Therefore, if Acanthamoeba is present in corneal scrapings or ocular secretions of AK patients, it is believed that Acanthamoeba can be sufficiently detected with this antibody.
Over 20 species of Acanthamoeba have been identified, of which, 8 species (A. castellanii, A. polyphaga, A. royreba, A. culbertsoni, A. hachetti, A. griffin, A. quina, and A. lugdunensis) could cause keratitis, with A. castellanii and A. polyphaga being the most common causes of AK [22]. In the present study, we used 6 species of Acanthamoeba (A. castellanii Castellani, A. castellanii Neff, A. hatchetti, A. polyphaga, A. culbertsoni, and A. royreba). Immunocytochemistry results revealed that the ACAP antibody reacted with all these Acanthamoeba spp (Figs. 4, 5). It would be nice to add the results of detecting A. griffin, A. quina, and A. lugdunensis by ACAP antibody. In addition, it is needed that the AK mouse model or human corneal scrapes can be used to detect Acanthamoeba spp. in future studies.
In summary, we generated a polyclonal peptide antibody against the ACAP protein of A. castellanii that specifically detected 6 species of Acanthamoeba trophozoites and cysts. This result suggests its potential for developing a diagnostic method for AK.
ACKNOWLEDGMENT
This work was supported by the National Research Foundation of Korea (NRF) grant funded by Korea government (MIST) (No. 2020R1A2C1005345).
Notes
The authors declare no conflict of interest related to this study.