Molecular Markers for Sulfadoxine/Pyrimethamine and Chloroquine Resistance in Plasmodium falciparum in Thailand
Article information
Abstract
Drug resistance is an important problem hindering malaria elimination in tropical areas. Point mutations in Plasmodium falciparum dihydrofolate reductase (Pfdhfr) and dihydropteroate synthase (Pfdhps) genes confer resistance to antifolate drug, sulfadoxine-pyrimethamine (SP) while P. falciparum chloroquine-resistant transporter (Pfcrt) genes caused resistance to chloroquine (CQ). Decline in Pfdhfr/Pfdhps and Pfcrt mutations after withdrawal of SP and CQ has been reported. The aim of present study was to investigate the prevalence of Pfdhfr, Pfdhps, and Pfcrt mutation from 2 endemic areas of Thailand. All of 200 blood samples collected from western area (Thai-Myanmar) and southern area (Thai-Malaysian) contained multiple mutations in Pfdhfr and Pfdhps genes. The most prevalent haplotypes for Pfdhfr and Pfdhps were quadruple and double mutations, respectively. The quadruple and triple mutations of Pfdhfr and Pfdhps were common in western samples, whereas low frequency of triple and double mutations was found in southern samples, respectively. The Pfcrt 76T mutation was present in all samples examined. Malaria isolated from 2 different endemic regions of Thailand had high mutation rates in the Pfdhfr, Pfdhps, and Pfcrt genes. These findings highlighted the fixation of mutant alleles causing resistance of SP and CQ in this area. It is necessary to monitor the re-emergence of SP and CQ sensitive parasites in this area.
INTRODUCTION
Although malaria is an ancient disease caused by Plasmodium parasite, it remains important to public health to present era. Plasmodium falciparum infection causes variable clinical symptoms ranging from asymptomatic to severe manifestations. The emergence of resistance of P. falciparum to the available antimalarial drugs is an important factor for malaria control [1]. In Thailand, resistance to many antimalarial drugs, including chloroquine (CQ), sulfadoxine-pyrimethamine (SP), mefloquine, and artemisinin has been reported [2,3]. CQ resistant P. falciparum was reported in the early 1960s [4,5]. In 1973, SP replaced CQ as the first-line treatment for uncomplicated falciparum malaria due to widespread resistance [1,6], but after 10 years, SP was ineffective [1,7]. Then, mefloquine was introduced in 1985 and resistance emerged in the same decade [8]. Artemisinin-based combination therapy was introduced as first-line treatment in 1995 [9].
Molecular epidemiological investigation provides information for detecting the emergence and spread of antimalarial drug resistance. Mutations in the P. falciparum dihydrofolate reductase (Pfdhfr) and P. falciparum dihydropteroate synthase (Pfdhps) genes (at codons 51, 59, 108, and 164 of Pfdhfr and 437, 540, and 581 of Pfdhps) are associated with SP treatment failures [10,11]. The mutations in Pfdhfr and Pfdhps genes were staged, resulting in increased levels of SP drug resistance [12]. The P. falciparum CQ resistance transporter gene (Pfcrt) K76T mutation has been linked to P. falciparum CQ resistance [13].
In some countries, the withdrawal of CQ for P. falciparum treatment, Pfcrt mutation (K76T) gently decreased and disappeared completely [14–17]. Similar to withdrawal of SP for P. falciparum treatment, Pfdhfr and Pfdhps gene mutations also decreased in some countries [18–21]. Conversely, alleles conferring CQ and SP resistance still occur at high frequency after discontinuation of these drugs [22,23]. However, declining of Pfdhfr, Pfdhps, and Pfcrt mutations might be associated with duration of drug withdrawal and geographical differences.
The objective of the present study was to investigate the prevalence of 5 Pfdhfr (A16V, N51I, C59R, S108N/T, and I164L), 5 Pfdhps (S436A, A437G, K540E, A581G, and A613S/T) and 1 Pfcrt (K76T) mutation from 2 different endemic areas of Thailand.
MATERIALS AND METHODS
Ethics approval
The study protocol was reviewed and approved by the Ethics Committee of Thammasat University (COA No. 134/2561). All patients were informed about the study objectives, sampling technique, and the benefits of the study. Informed consents were obtained according to the ethical standards from all patients.
Sample collection
A total of 200 dried blood spot samples were collected during 2007–2017 from patients with P. falciparum infection who attended malaria clinics in the western (Tak Province) and southern (Yala Province) regions along the Thai-Myanmar and Thai-Malaysian border, respectively.
Extraction of parasite genomic DNA
Genomic DNA of all blood samples was prepared using a QIAamp DNA extraction mini-kit (QIAGEN, Valencia, California, USA) according to manufacturer’s instruction and used as a template for polymerase chain reaction (PCR) amplification.
Amplification and detection of the Pfdhfr and Pfdhps
Pfdhfr and Pfdhps genes were amplified by nested PCR using Pfdhfr and Pfdhps specific primers (Table 1) according to the previously described methods with some modification [24]. Briefly, the PCR was carried out with the following reaction mixture including 0.25 μM of each primer, 1.5 mM MgCl2 (Thermo scientific, Waltham, Massachusetts, USA), 1×Taq buffered with KCl (Thermo scientific), 200 μM deoxynucleotides (dNTPs) (Bioline, London, UK), 2 μl of genomic DNA in the primary PCR, and 1 μl of primary PCR product in nested PCR and 1 unit of Taq DNA polymerase (Thermo scientific). All of the PCR products were then analyzed on 1% agarose gel and visualized under UV illuminator. PCR products were digested with restriction enzymes (Table 1) [24] then the restriction fragments were analyzed on 1.2% agarose gel and visualized under UV illuminator.
Amplification and detection of the Pfcrt
Amplification of K76T was performed by nested PCR using Pfcrt specific primers (Table 1) according to the previously described methods with some modification [25–27]. Briefly, the PCR was carried out with the following reaction mixture including 0.1 μM of each primer, 2.5 mM MgCl2 (Thermo scientific), 1×Taq buffered with KCl (Thermo scientific), 100 μM deoxynucleotides (dNTPs) (Bioline), 0.5 μl of genomic DNA in the primary PCR, and 0.5 μl of primary PCR product in nested PCR and 0.5 unit of Taq DNA polymerase (Thermo scientific). All of the PCR products were then analyzed on 1.5% agarose gel and visualized under UV illuminator. PCR products were digested with restriction enzymes ApoI (New England Biolabs Inc., Hertfordshire, UK) (Table 1), as described by the manufacturer. Then the restriction fragments were analyzed on 2.0% agarose gel and visualized under UV illuminator.
Statistical Analysis
Data analysis was performed by SPSS software version 21.0 (IBM Corporation, Armonk, New York, USA). The chi-square test was used to compare the frequencies and correlations of all data. The level of significance was set at P<0.05.
RESULTS
Analysis of Pfdhfr and Pfdhps mutations
A total of 200 P. falciparum samples were successfully amplified and analyzed for both the Pfdhfr and Pfdhps genes. The polymorphisms at each codon were demonstrated by restriction fragments (Fig. 1A, B). The frequencies of Pfdhfr and Pfdhps mutations was summarised in Table 2. All samples had at least 1 codon mutation in the Pfdhfr (A16V, N51I, C59R, S108N/T, and I164L) and Pfdhps (S436A, A437G, K540E, A581G, and A613S/T). Four codon mutations were detected in Pfdhfr (51I, 59R, 108N, and 164L) in samples from 2 areas. All isolates carried mutations at codon 59 and 108 in Pfdhfr. Four codon mutations were detected in Pfdhps (436A, 437G, 540E, and 581G) in samples from Tak Province, whereas 3 codon mutations were detected from Yala Province. All isolates carried wild-type alleles at codon 613 in Pfdhps. Mixed genotypes were detected in thirty isolates by codon 51 and 164 of Pfdhfr, and 436 and 540 of Pfdhps that were no processed further. There was no wildtype allele ANCSI, but 3 alleles (AIRNI, AIRNL, and ANRNL) of Pfdhfr were identified in this study (Table 3). AIRNL was the most prevalent allele (52.4%) in all P. falciparum isolates collected from Tak Province (88.9%) (Fig. 2). AIRNI was the most prevalent allele in P. falciparum isolates from Yala Province (80.9%). Statistical significance was found between 2 study areas (P<0.001). For Pfdhps, 6 alleles were identified (Table 3). SGKGA was the most prevalent allele (50.0%) found in P. falciparum isolates from Yala Province (83.1%). SGEGA was the most prevalent allele in P. falciparum isolates from Tak Province (70.4%). Statistical significance was found between 2 study areas (P<0.001). Eleven allele combination of Pfdhfr-Pfdhps were found in this study (Table 4). The quintuple mutation (AIRNI-SGKGA), which comprise triple mutations in Pfdhfr and 2 mutations in Pfdhps were found to be most prevalent in study population (35.3%) and in isolates from Yala Province (66.3%). The septuple mutation (AIRNL-SGEGA), which comprised quadruple mutations in Pfdhfr and 3 mutations in Pfdhps were most frequent in isolates from Tak Province (64.2%).
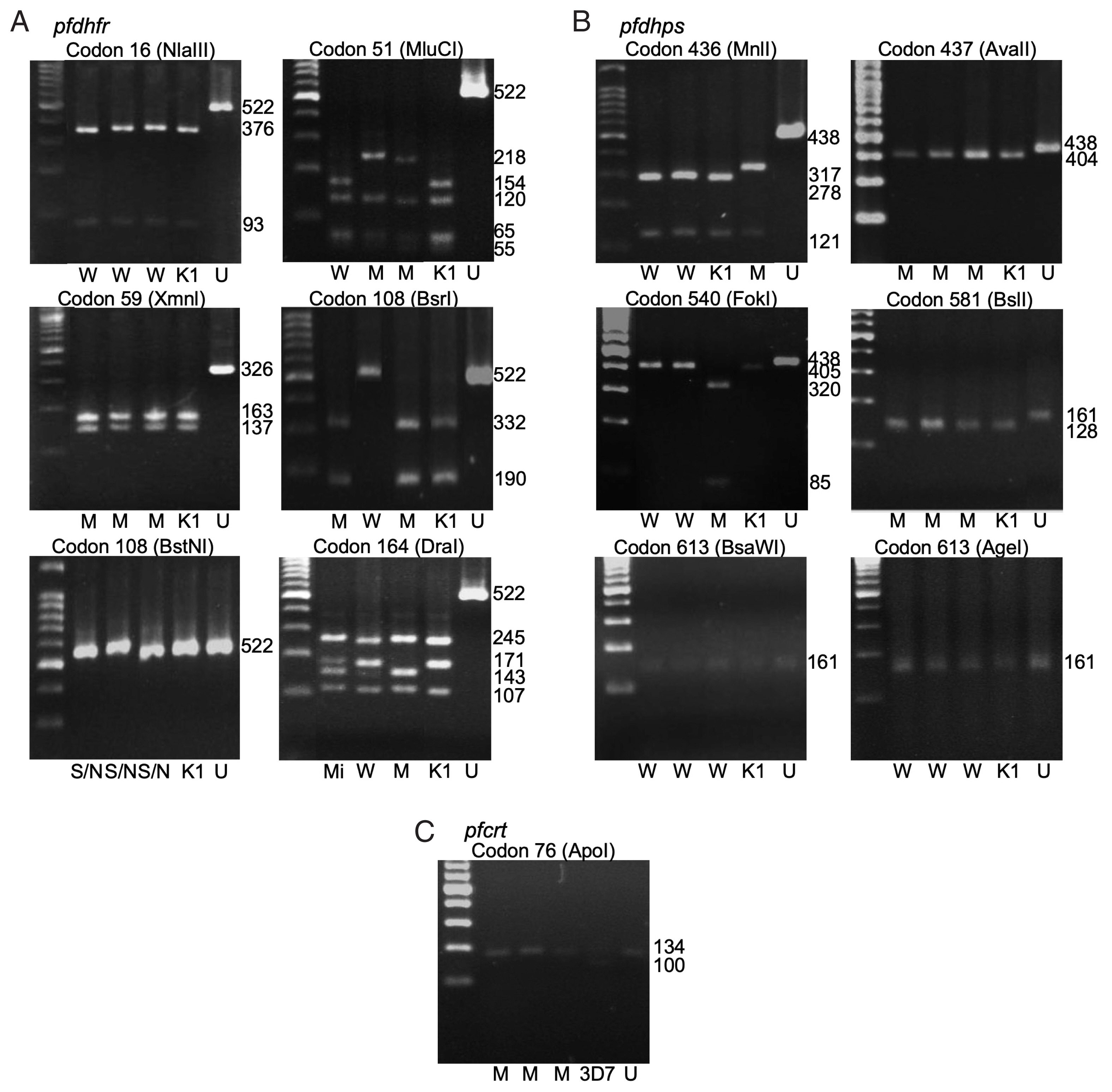
The polymorphism of pfdhfr (A), pfdhps (B), and pfcrt (C) gene by gel electrophoresis. W-wildtype, M-mutant, S/N-serine/threonine, Mi-mixed, K1-P. falciparum K1 strain, 3D7- P. falciparum 3D7 strain, U-undigested fragment. Fragment sizes in base pair (bp) are shown.
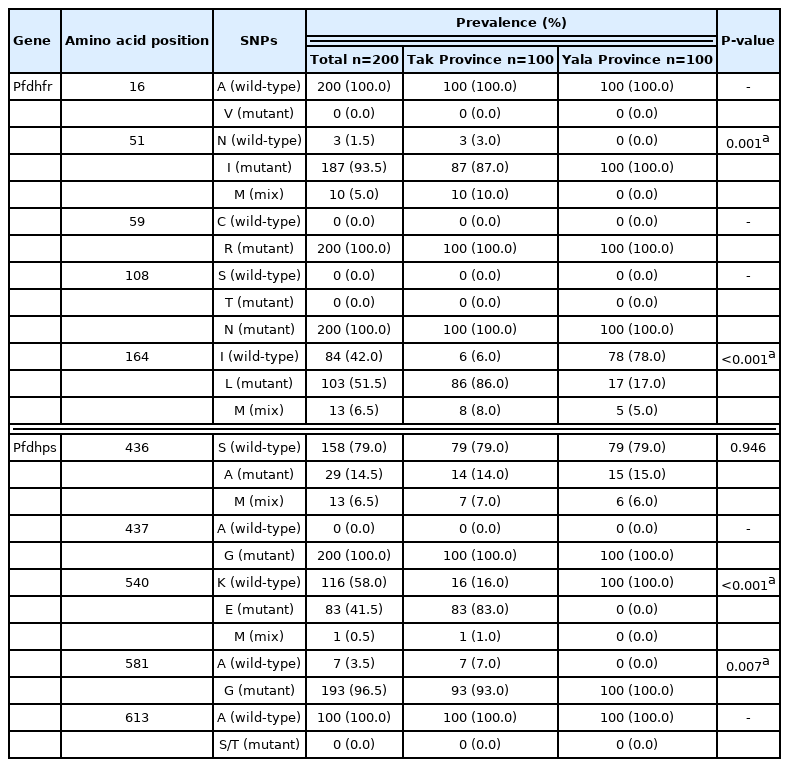
Prevalence of Plasmodium falciparum dihydrofolate reductase (Pfdhfr) and dihydropteroate synthase (Pfdhps) single nucleotide polymorphisms (SNPs) in 200 P. falciparum isolates from 2 endemic areas of Thailand
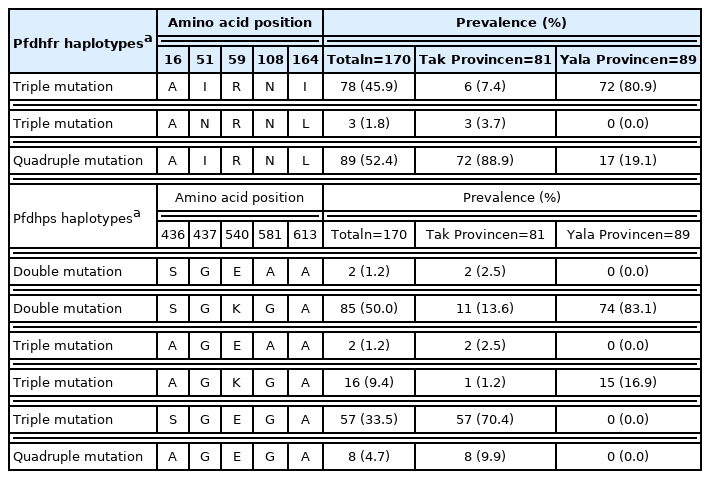
Plasmodium falciparum dihydrofolate reductase (Pfdhfr) and dihydropteroate synthase (Pfdhps) alleles in 170 P. falciparum isolates from 2 endemic areas of Thailand
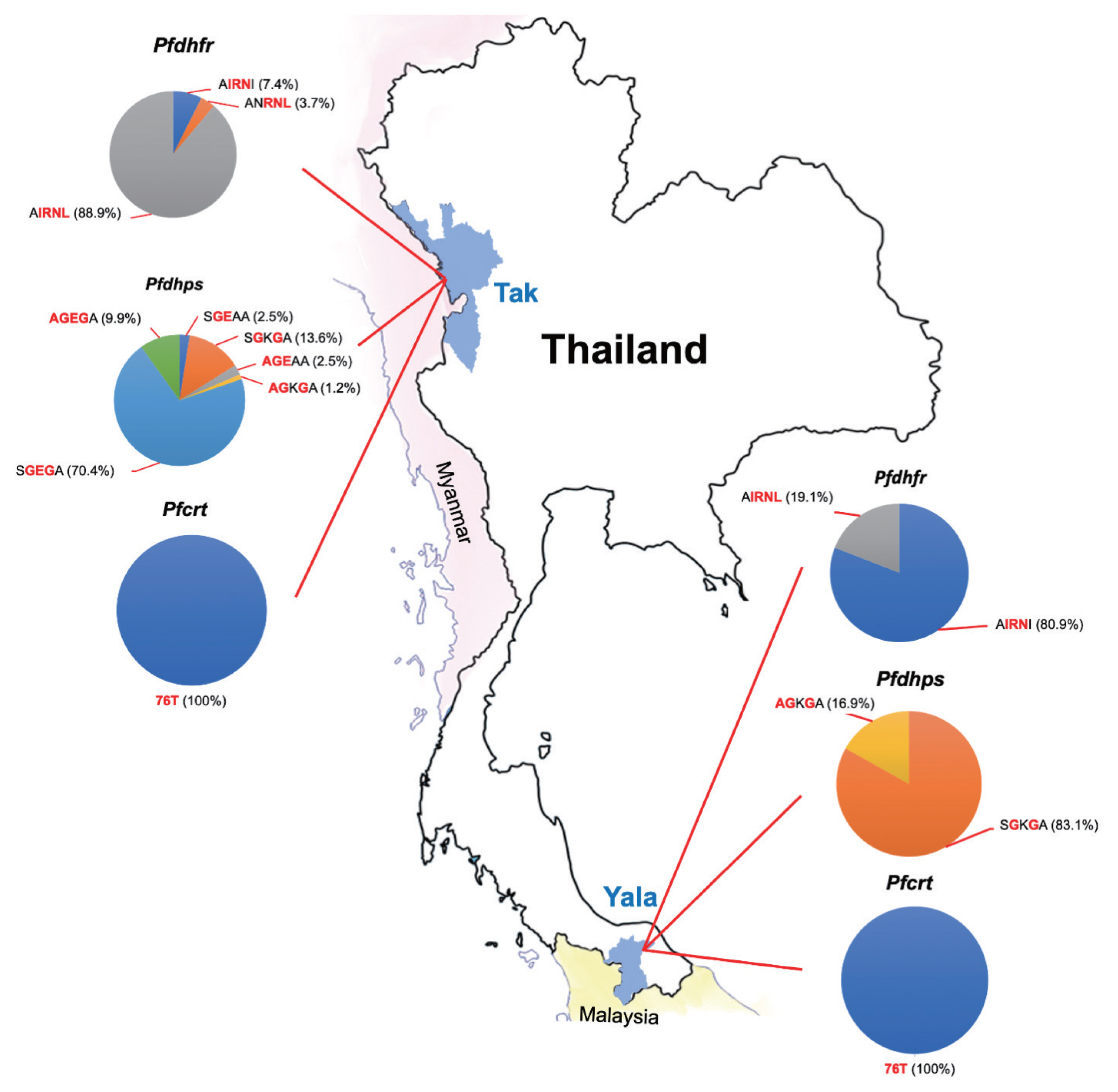
The proportions of mutations in 3 resistance genes (pfdhfr, pfdhps, and pfcrt) observed in P. falciparum isolates in this study.
DISCUSSION
Mutations on Pfdhfr and Pfdhps genes associated with SP resistance have been reported in several malaria endemic areas such as Guinea [28], Indonesia [29], Malaysia [30], Myanmar [31], and Thailand [32,33]. In Thailand, a previous study revealed that the change of Pfdhfr point mutations from double mutations to triple and quadruple mutations in some areas [34,35] which the number of mutations is correlated with increased level of SP resistance [12]. In the present study, all P. falciparum isolates had at least three mutation point in Pfdhfr genes, indicated persistence of highly mutations on SP resistant markers. The Pfdhps mutation studies conducted between 2001 and 2007 in Thailand indicated that there has been fluctuation of the Pfdhps mutations between triple and quadruple mutations. In this study, predominant frequency of double mutation was found especially in isolates from southern endemic area. The predominance of triple mutations was also found in isolates from western area. This result indicated that high mutation in Pfdhfr and Pfdhps genes with different frequency existed in these 2 different localities. In Thailand, SP was withdrawn from P. falciparum treatment for many years. Although decreased of Pfdhfr and Pfdhps mutations were reported from some countries after the withdrawal of these drugs, high frequency of Pfdhfr and Pfdhps mutations still present in Thailand. This existence of mutations on Pfdhfr and Pfdhps genes may be associated with using of other antifolate drugs that can also induce pressure on Pfdhfr and Pfdhps.
A previous study has demonstrated that high prevalence of Pfcrt K76T mutation in study isolates might contribute to CQ resistance to P. falciparum [36]. A high prevalence rate of Pfcrt K76T mutation was previously observed in several countries such as Mali [25], Kenya [37], Indonesia [26], Philippines [38] and Thailand [39–41]. Declining of Pfcrt mutations after withdrawal of CQ has been reported in Malawi [14], Tanzania [15], Kenya [16], and China [17]. However, even CQ was withdrawn from Thailand for long period, the CQ resistance allele still remains with high frequency. This complete fixation of CQ resistance in P. falciparum is might due to the co-existence of P. falciparum and P. vivax infections in this country while CQ is a standard regimen for P. vivax malaria treatment, leading to the phenomenon of continuous exposure to drug pressure in P. falciparum.
Our study demonstrates a high prevalence of Pfdhfr, Pfdhps, and Pfcrt mutations of P. falciparum isolates from 2 endemic areas in Thailand, emphasizing the fixation of mutant Pfdhfr, Pfdhps and Pfcrt alleles that confer consistent resistance of SP and CQ. SP and CQ drugs are still not appropriate for P. falciparum treatment in Thailand and other antimalarial groups should be considered.
ACKNOWLEDGMENT
This research was supported by the Program Management Unit for Human Resources and Institutional Development, Research and Innovation, NXPO [grant number B05F630043].
Notes
The authors have no conflict of interest.