An Evaluation of a New Quantitative Point-of Care Diagnostic to Measure Glucose-6-phosphate Dehydrogenase Activity
Article information
Abstract
Malaria continues to be one of the most crucial infectious burdens in endemic areas worldwide, as well as for travelers visiting malaria transmission regions. It has been reported that 8-aminoquinolines are effective against the Plasmodium species, particularly primaquine, for anti-hypnozoite therapy in P. vivax malaria. However, primaquine causes acute hemolytic anemia in individuals with glucose-6-phosphate dehydrogenase (G6PD) deficiency. Therefore, G6PD deficiency testing should precede hypnozoite elimination with 8-aminoquinoline. Several point-of-care devices have been developed to detect G6PD deficiency. The aim of the present study was to evaluate the performance of a novel, quantitative G6PD diagnostics based on a metagenomic blue fluorescent protein (mBFP). We comparatively evaluated the sensitivity and specificity of the G6PD diagnostic modality with standard methods using 120 human whole blood samples. The G6PD deficiency was spectrophotometrically confirmed. The performance of the G6PD quantitative test kit was compared with that of a licensed control medical device, the G6PD strip. The G6PD quantitative test kit had a sensitivity of 95% (95% confidence interval (CI): 89.3–100%) and a specificity of 100% (95% CI: 94.3–100%). This study shows that the novel diagnostic G6PD quantitative test kit could be a cost-effective and time-efficient, and universally mandated screening tool for G6PD deficiency.
INTRODUCTION
Glucose-6-phosphate dehydrogenase (G6PD) is a key enzyme in the pentose phosphate pathway, which converts NADP+ into NADPH during the oxidation of glucose-6-phosphate. NADPH acts as a reducing agent to produce reduced glutathione, which protects cells against harmful oxidative metabolites [1]. In particular, G6PD is the only source of NADPH production in red blood cells. G6PD is considered a key enzyme for cell survival and the maintenance of intracellular redox equilibrium.
G6PD deficiency is an inherited enzymatic disorder causing hemolytic anemia and follows an X-linked recessive inheritance pattern in humans by loss-of-function, which affects over 400 million people globally and is one of the most polymorphic proteins with at least 140 mutations identified [2,3]. The study of G6PD deficiency in relation to the use of drugs is a classic example of pharmacogenetics [4]. G6PD deficiency-the most common human enzymatic deficiency-causes destruction of red blood cell as observed in several infections and the intake of fava beans, and numerous drugs, including aspirin, nitrofurantoin, quinidine, quinine, and sulfa drugs [5,6]. G6PD deficiency has a prevalence of 0.1–30% in Southeast Asia, Africa, and the Mediterranean, which varies among ethnic groups in different geographic regions [3,7]. The rate of G6PD deficiency in Korea is about 0.9–3.5% [8,9], while it is close to or more than 5% throughout the Asia Pacific region [10]. G6PD is also involved in the pathogenesis of several diseases, including heart failure, type 2 diabetes, and hypertension. The distribution of G6PD deficiency correlates with the malaria-endemic areas [11], showing the evolutionary selection of G6PD deficiency by malaria [12]. Recently, it was reported that the expression and activity of G6PD in tumor tissues is higher than in normal cells, and that inhibition of G6PD expression in tumor cells induces apoptosis and reduces proliferation. In addition, since p53, a tumor suppressor, inhibits PPP and G6PD activity through binding to G6PD and the inhibition of active dimer formation, G6PD is a possible therapeutic target protein for tumors. Erythrocytes exposure to oxidative stress causes hemoglobin denaturation, ultimately resulting in hemolysis. Thus, G6PD deficiency can lead to hemolytic anemia with jaundice. Hemolytic anemia in G6PD-deficient individuals can be triggered by a range of oxidative agents, such as infections and certain food and drugs, including anti-malaria drugs (e.g., primaquine) [13,14]. Thus, G6PD can be used not only as diagnostic tool but also a therapeutic target. Therefore, it is essential to develop a sensitive, high-throughput assay for the quantitative analysis of G6PD activity [15]. Although a great deal is well understood, determining the potential for drug-induced hemolysis in the clinical setting is still challenging. Primaquine, an 8-aminoquinoline, is the only drug approved by the World Health Organization (WHO) to treat the hypnozoite stage of Plasmodium vivax and P. ovale malaria [16]. Relapse, triggered by the activation of dormant hypnozoites in the liver, can occur weeks to years after primary infection, and is the predominant source of transmission in endemic settings. Hence, primaquine is essential for individual treatment and elimination of P. vivax. However, use of primaquine is limited by the risk of life-threatening acute hemolytic anemia in G6PD-deficient individuals.
As G6PD testing is not generally accessible in most malaria-endemic regions of the world, lower dosages of primaquine are recommended to reduce the risk for suspected G6PD-deficient patients [17]. However, G6PD-deficient patients have been consistently increased, and G6PD deficiency-mediated hemolytic anemia patients was reported in the ROK Army, after primaquine uptake without G6PD testing [18,19]. In addition, there has been a recent increase in the number of multicultural families due to the increase in immigrants from countries, where Plasmodium malaria species circulate and the frequency of G6PD deficiency is as high as 30%. These families constitute 4.3% of the total population in Korea and are fast approaching 5%, classifying Korea as a multicultural country [20]. Moreover, the number of second-generation children with G6PD deficiency is expected to grow with the increase in the number of multicultural families in Korea since the mid-1990s being driven by a large number of international marriages [21] and foreigners with Korean citizenship [22]. Although international migration has become a global phenomenon over the past decades, this has occurred only relatively recently in Korea. Among the registered foreign residents in 2018, 6.7% (159,206/2,367,607) of them were marriage migrants, the second largest group, followed by non-professional migrant workers [23]. Approximately 83% (132,391/159,206) of international marriages were between a Korean groom and a foreign bride, most of whom are from East and Southeast Asian countries [24]. Thus, G6PD testing is necessary because there are now many ethnic groups in Korea.
The purpose of this study was to evaluate the sensitivity and specificity of the medical device, G6PD quantitative test kit, which helps in diagnosing G6PD deficiency by quantifying the activity of G6PD in human whole blood by clinical chemical method. This quantitative test is designed to discriminate G6PD deficiency from those with normal enzyme activity, usually at the cut-off threshold of 30% of normal activity. G6PD testing must precede hypnozoite elimination. To date, such tests are not commercially available to vulnerable populations, especially at the community level for residents of tropical areas with prevalent P. vivax malaria, who are most in need. To overcome the technical difficulty in the field and even at major clinics and hospitals, various point-of care devices for G6PD deficiency have been developed in recent years for a rapid screening procedure [25–28]. However, these tests have some inherent limitations. There are several issues to be considered when assessing what technology should be included when testing is implemented [25]. To analyze G6PD deficiency prevalence and malaria chemoprophylaxis risk, the development of point-of-care screening tests for G6PD deficiency, which is a cost-effective and time-efficient, and universally mandated, will be required for use in malaria case management.
MATERIALS AND METHODS
Ethics statement and sample collection
This study was reviewed and approved by the Institutional Review Board of the Inha University Hospital (Approval No. 2020-09-005) and conducted according to the principles of the 1964 Helsinki Declaration. A total of 120 residual blood samples that met the subject selection criteria were collected from the Inha University Global Resource Bank of Parasitic Protozoa Pathogens. The clinical trial was performed in a randomized, single-blind, and retrospective manner for the evaluating the clinical sensitivity, specificity, and correlation (concordance rate) of medical devices using human whole blood samples. Because the prevalence of G6PD deficiency in Korea is very low and G6PD deficiency is an X-linked recessive hereditary disorder, this study was conducted on multicultural families including immigrant mothers and their second-generation children. The average age of these 120 subjects (80 women and 40 men) was 24.2 years (range, 2–60), which were collected from June 2020 to May 2021. Malaria infections were confirmed by microscopic examination and nested PCR. Specimens used in this trial were anonymized and randomized so that investigators had no information about the specimens. G6PD activity in human blood samples was determined spectrophotometrically and calibrated with deficient, intermediate, and normal controls provided by the kit (Trinity Biotech, Wicklow, Ireland). To ensure precise evaluation, all 120 whole blood samples were stored at 4°C for less than 7 days. Both the whole blood specimens and controls were treated by the same procedure. Spectrometry results of these individuals collected during this study were used to define the median enzyme activity, using G6PD strip (CareSTART S1 G6PD Strip, Wells Bio, Seoul, Korea), G6PD quantitative test kit (DiaRapid, SolGent, Daejeon, Korea), and spectrophotometry, and defined as 100% G6PD activity for each test (11.5 IU/g hemoglobin (IU/g Hb)). The G6PD activity of all individuals enrolled in this study was then calculated as a percentage of the median value for each test.
Procedure
We determined the sensitivity and specificity of G6PD quantitative test kit using the residual whole blood sample of patients with G6PD deficiency confirmed by comparing the results with those of a comparative diagnostic reagent (Glucose-6-Phosphate Dehydrogenase Quantitative Test, Trinity Biotech) and comparative evaluation with a control medical device “G6PD strip (S1 G6PD Strip)” according to the manufacturers’ instructions. The reliability of the G6PD quantitative test (Trinity Biotech) was monitored by calibration and by including 3 different controls provided by Trinity Biotech (Deficient level: reference G5888; intermediate level: reference G5029; and normal level: reference G6888) in each test run. The hemoglobin level was measured by using the Hb 201 analyzer (HemoCue AB, Ängelholm, Sweden) to normalize G6PD enzyme activity for hemoglobin. G6PD enzyme activity measured by G6PD strip was expressed as International Units/dl (IU/dl). G6PD enzyme activity was normalized for hemoglobin values measured at the same time as G6PD measurement and expressed as IU/g Hb.
For the G6PD quantitative test kit, irradiation with light near 350 nm, which showed the maximum fluorescence through excitation scanning. Hence, after irradiation with light near 350 nm, the relative value of G6PD was measured using the G6PD quantitative test kit at the emission wavelength (450 nm) to determine the amount corresponding to the standard curve. The test kit with the G6PD quantitative test kit allowed the simultaneous quantitative measurement of red cell G6PD activity (IU/g Hb) and hemoglobin level in finger-stick (capillary) or venous whole blood (EDTA anticoagulated) without normalizing to the value of the hemoglobin amount of the same blood volume used to measure the G6PD activity. For quantitative analysis, 2 μl of whole blood were added to the test buffer (650 μl) and mixed. The blood-buffer mixture was then added to the test chamber of the G6PD quantitative test kit. The analyzed results were provided as the digitalized value on the G6PD quantitative test kit panel.
Statistical analysis
G6PD measurements recorded by the G6PD strip and G6PD quantitative test kit were compared with the reference method spectrometry using Pearson correlation (r) or Spearman’s rank correlation (rs), as appropriate and Bland-Altman plots.
All G6PD results were categorized as a percentage of the median value (11.5 IU/g Hb) from the previous report [21,29] according to the current threshold used to determine exclusion from primaquine treatment (<30% activity) [30].
RESULTS
A total of 120 individuals were tested in this study. None of participants had malaria infection. The participants were predominantly female (80, 66.7%), with a mean Hb concentration of 12.14 g/dl, as determined by the Hb 201 analyzer machine. As a result of testing 120 residual whole blood samples were selected according to the selection criteria using a medical device for comparable diagnostic reagent testing. The median value of a normal adult male in this study was 11.5 IU/g Hb and this value was used to define 100% G6PD activity [21]. According to WHO’s G6PD testing guidelines, values less than 10%, 10 to <30%, and 30 to <60% of the median are defined as severe, moderate, and mild deficient (normal range: 6.9–16.3 IU/g Hb), respectively. The 100 percent of G6PD activity was adjusted to 11.5 IU/g Hb as previously reported [21]. Twelve (10.0%) participants had less than 10% G6PD activity (<1.2 IU/g Hb), 48 (40.0%) had between 10% and 30% (≥1.2 IU/g Hb and <3.5 IU/g Hb), 18 (15.0%) had activities between 30% and 60% (≥3.5 IU/g Hb and <6.9 IU/g Hb) and 42 (35.0%) participants had G6PD activities greater than 60% (≥6.9 IU/g Hb). When 30% (<3.5 IU/g Hb) of the median was applied and compared with the results confirmed at the time of selection, 60 positive and 60 negative samples were confirmed using the spectrophotometry test as a gold standard based on the enzyme kinetics [29] (Fig. 1).
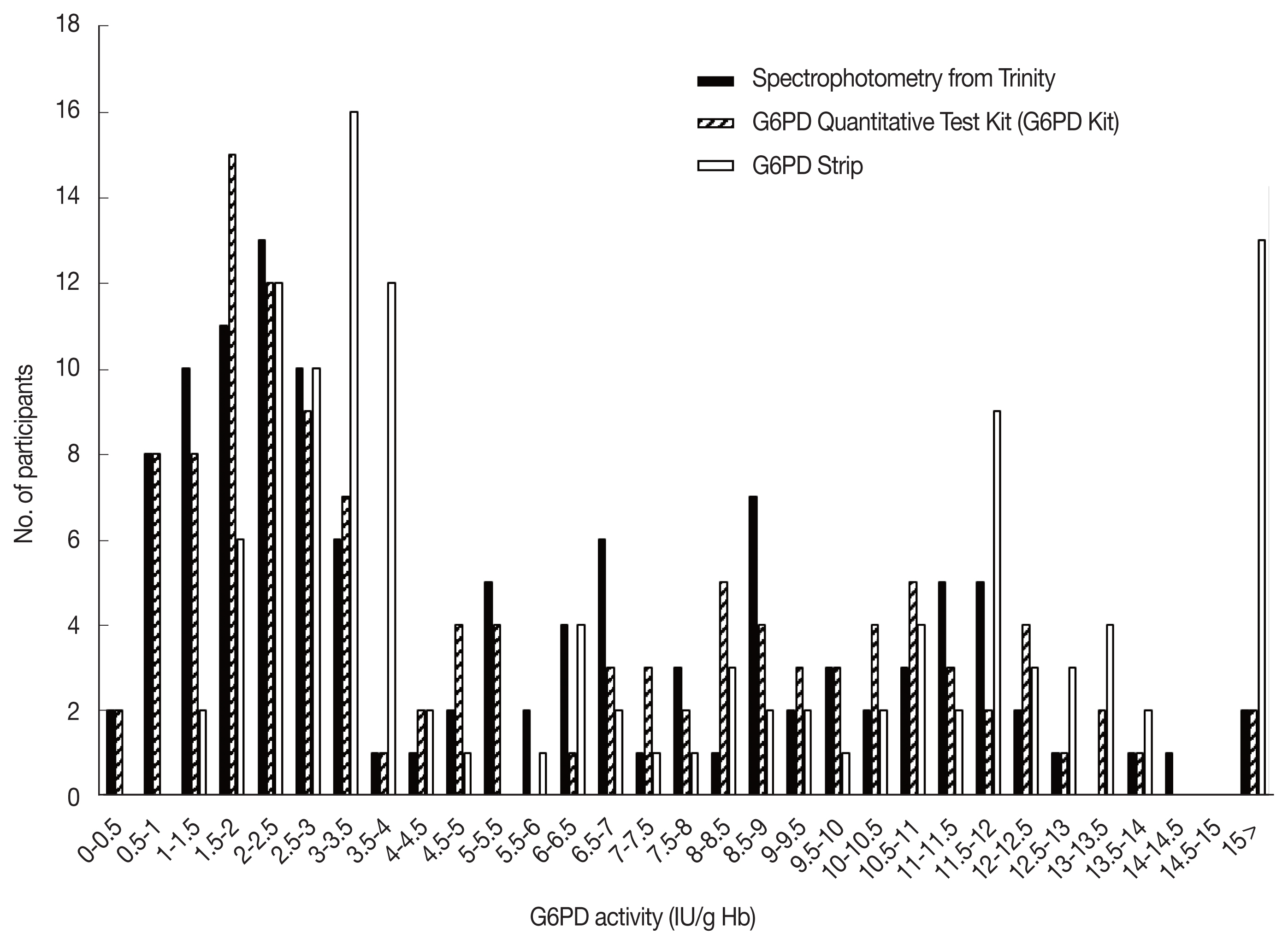
Distribution of the G6PD enzymatic activity (IU/g Hb) values of 120 blood samples. Activity measurement was performed on the blood samples using the quantitative G6PD strip (Black bars), G6PD quantitative test kit (Striped bars), and G6PD Strip (Open bars).
Comparing spectrophotometry with the G6PD quantitative test kit while considering less than 30% of the median value (3.5 IU/g Hb), 57 out of 60 positive whole blood samples were judged to be positive and 3 were as negative, resulting in a clinical sensitivity of 95% (57/60) (95% confidence interval (CI): 89.3–100%) using the G6PD quantitative test kit (Table 1). The negative whole blood samples from 60 cases were all negative (specificity of 100%, 95% CI: 94.3–100%). The G6PD quantitative test kit resulted in a positive predictive value (PPV) of 100% (n=60/60) and negative predictive value (NPV) of 95.2% (n=60/63). Compared to spectrophotometry, the G6PD strip had sensitivity and specificity of 81.3% (95% CI: 80.6–94.4%) and 100% (95% CI: 94.3–100%), respectively (Table 1). The G6PD strip resulted in a PPV of 100% (n= 60/60) and NPV of 95.2% (n=60/63).
The correlation coefficient (rs) for activity measurements from the G6PD quantitative test kit and the spectrophotometry was 0.972 (P<0.001), with a mean difference of 0.05 IU/g Hb (Standard deviation (SD)=±1.36, Range: −2.61 to 2.71) (Fig. 2). For the G6PD strip and the spectrophotometry was rs was 0.906 (P<0.001) with a mean difference of 1.79 IU/g Hb (SD=±1.77, range: −1.68 to 5.26) (Fig. 3). The area under the receiver operator curve (AUC) was 0.975 (95% CI: 0.943–1.000) for the G6PD quantitative test kit and 0.875 (95% CI: 0.806–0.944) for the G6PD strip (Fig. 4).
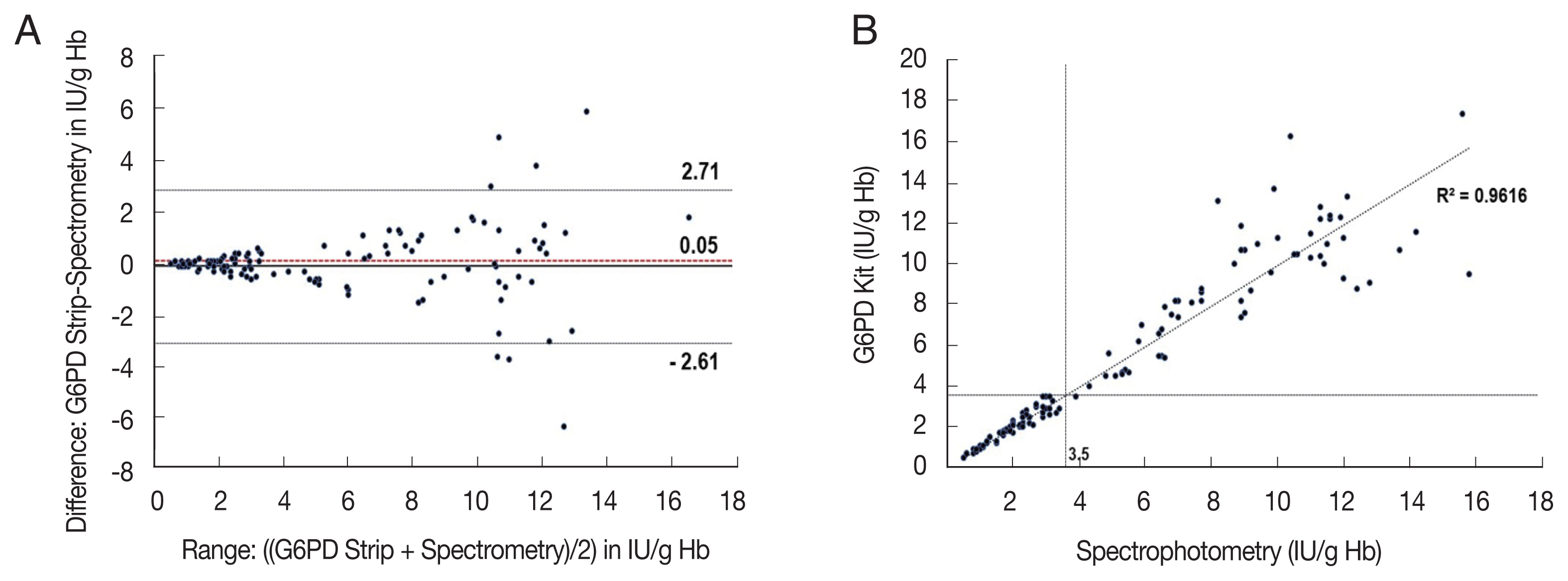
Comparison of the G6PD quantitative test kit with spectrophotometry (A) and scatter plot (B). (A) Mean difference: 0.05 IU/g Hb, 95% Limits of Agreement (LoA): −2.61 to 2.71 IU/g Hb (B) rs=0.972; P<0.001, n=120, dotted-lines indicate 30% threshold (3.5 IU/g Hb).
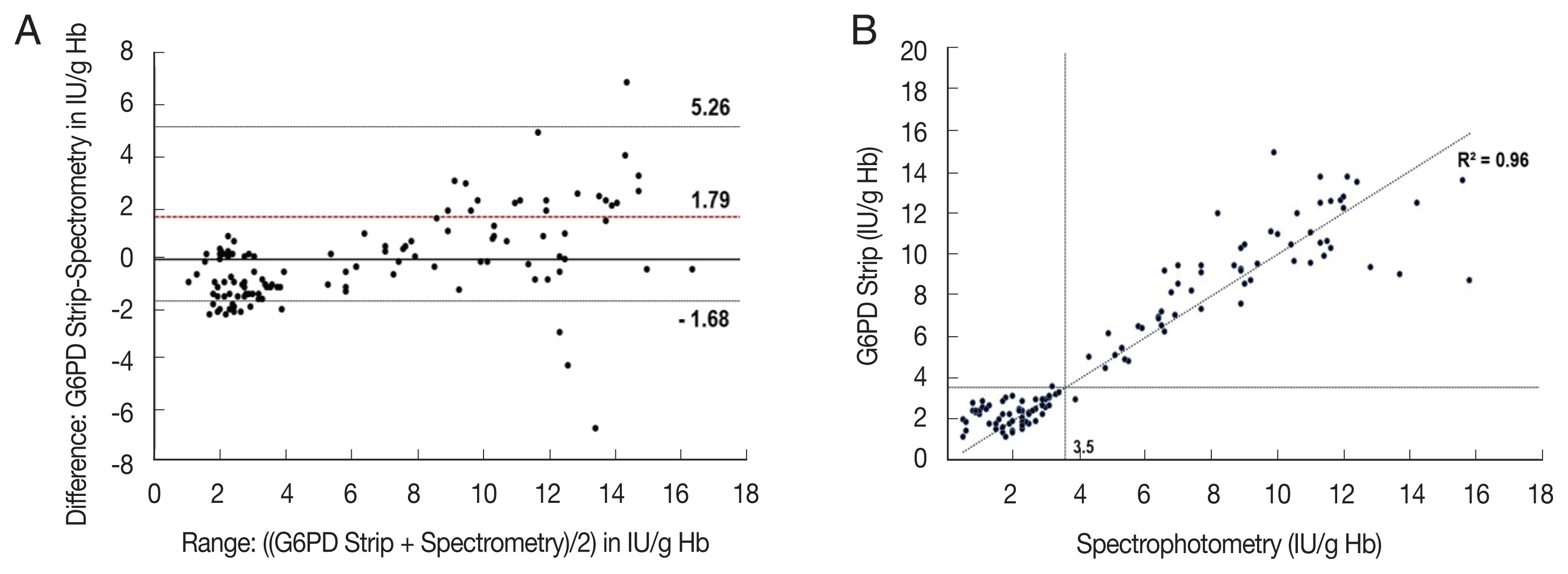
Comparison of the G6PD strip with spectrophotometry (A) and scatter plot (B). (A) Mean difference: 0.79 IU/g Hb, 95% LoA: −1.68 to 5.26 IU/g Hb. (B) rs=0.906; P<0.001, n=120, broken lines indicate 30% threshold (3.5 IU/g Hb).
DISCUSSION
Here, we present from a novel device to quantify G6PD activity at the point of care. The diagnostic performance of the device was compared with spectrophotometry and another rapid diagnostic test (RDT) kit, G6PD strip, which showed a high correlation with the reference spectrophotometry method. Various NADPH quantitative techniques have been used to diagnose G6PD deficiency, and the most common strategy is to measure absorbance or fluorescence using the intrinsic wavelength of NADPH itself. However, due to the low sensitivity, at least 5 min is required to detect significant changes in NADPH. The quantitative G6PD assay method used as the gold standard [31] is based on the enzyme kinetics-based spectrophotometric methods [32,33]. This involves the reduction of NADP to NADPH, by the G6PD enzyme in the presence of glucose-6-phosphate, measured spectrophotometrically at 340 nm. This method is the most accepted and sensitive assay to measure G6PD activity. Although this gold standard kit is most commonly used, it is labor-intensive and time consuming (Table 2). The G6PD strip (S1 G6PD strip) is based on the electrochemical principle of G6PD enzyme activity by measuring the reduction of NADPH coupled with the ferricyanide [31]. However, in this study the G6PD strip could not detect G6PD levels of <1.5 IU/g Hb. The G6PD quantitative test kit involves the formation of a metagenomic blue fluorescent protein (mBFP)-NADPH complex. mBFP from soil bacteria has no intrinsic fluorescence but shows significantly high blue fluorescence at 450 nm specifically on interaction with NADPH when excited at 350 nm [34,35]. Irradiating light near 350 nm, which shows the maximum fluorescence of this complex through excitation scanning. Hence, after irradiating light near 350 nm, the relative value at the emission wavelength (approximately 450 nm) is measured to determine the amount of NADPH corresponding to the standard curve. As these technologies do not require the addition of a separate substrate other than coenzyme, they have the advantage of simplicity, although the absorbance and fluorescence values of NADPH itself are low. The formation of the mBFP-NADPH complex causes an increase in the fluorescence intensity of NADPH by more than 10-fold. Thus, the use of this complex facilitated the development of a simple and inexpensive instrument for fluorescence measurement (G6PD quantitative test kit) and related reagents for the quantitative measurement of NADPH. The G6PD quantitative test kit showed an almost perfect correlation with spectrophotometry in the overall range, suggesting that the G6PD quantitative test kit has a comparable discriminatory power (Figs. 2, 4). The G6PD quantitative test kit is designed to simultaneously measure the fluorescence required to measure the G6PD activity and the absorbance required to measure the hemoglobin value. A significant change in NADPH amounts could be detected in just 1 min using this low-cost fluorescence measurement equipment. The G6PD quantitative test kit enables the quantitative analysis of G6PD activity in a short time. It can be used in underdeveloped countries lacking cooling facilities (Table 2). Quantitation of G6PD is suitable for G6PD measurement without separately measuring the hemoglobin level, thus can provide a rapid test result. It is an attractive alternative to the G6PD enzyme assay.
The results of the G6PD spectrophotometric assay as the gold standard for the G6PD deficiency test also support those of G6PD quantitative test kit in our study without hemoglobin measurement to be performed in parallel (Figs. 1, 2, 4). During the anemic phase caused by reticulocytosis or blood loss, G6PD activity does not reflect the baseline G6PD activity of the individual. Furthermore, the G6PD quantitative test kit was simple to use even in the field, with the sample collected directly from a finger-pick. It was reported that G6PD enzyme activity remained stable at room temperature but had decreased by 5% at 24 h. At 4°C, the activity did not fall by more than 10% until 9 days of storage. The G6PD quantitative test kit is operable under temperature conditions of any field.
In conclusion, our study presents a novel diagnostic method, which is based on a new scientific tool, the mBFP. It could perform well in laboratory conditions as well as in the field with comparable accuracy. It offers a reliable alternative to the gold standard spectrophotometry in settings with poor laboratory infrastructure and has the potential to facilitate the strong and safe roll out of the radical treatment of P. vivax malaria.
ACKNOWLEDGMENTS
This study was supported by funding from the National Research Fund (NRF-2020R1F1A1070882, and NRF-2022R1F1 A1066481) in the Republic of Korea, and the Bio and Medical Technology Development Program of the NRF funded by the Korean Government (MSIT) (2018M3A9H5055614).
Notes
The authors declare that they have no conflicts of interest.