Evaluating the activity of N-89 as an oral antimalarial drug
Article information
Abstract
Despite the recent progress in public health measures, malaria remains a troublesome disease that needs to be eradicated. It is essential to develop new antimalarial medications that are reliable and secure. This report evaluated the pharmacokinetics and antimalarial activity of 1,2,6,7-tetraoxaspiro[7.11]nonadecane (N-89) using the rodent malaria parasite Plasmodium berghei in vivo. After a single oral dose (75 mg/kg) of N-89, its pharmacokinetic parameters were measured, and t1/2 was 0.97 h, Tmax was 0.75 h, and bioavailability was 7.01%. A plasma concentration of 8.1 ng/ml of N-89 was maintained for 8 h but could not be detected at 10 h. The dose inhibiting 50% of parasite growth (ED50) and ED90 values of oral N-89 obtained following a 4-day suppressive test were 20 and 40 mg/kg, respectively. Based on the plasma concentration of N-89, we evaluated the antimalarial activity and cure effects of oral N-89 at a dose of 75 mg/kg 3 times daily for 3 consecutive days in mice harboring more than 0.5% parasitemia. In all the N-89- treated groups, the parasites were eliminated on day 5 post-treatment, and all mice recovered without a parasite recurrence for 30 days. Additionally, administering oral N-89 at a low dose of 50 mg/kg was sufficient to cure mice from day 6 without parasite recurrence. This work was the first to investigate the pharmacokinetic characteristics and antimalarial activity of N-89 as an oral drug. In the future, the following steps should be focused on developing N-89 for malaria treatments; its administration schedule and metabolic pathways should be investigated.
Introduction
Malaria is a global health concern severely affecting the developing countries responsible for significant morbidity and mortality cases in malaria-endemic regions. It is estimated that around 619,000 people die from malaria annually [1]. Recently, several new antimalarial drugs or their combinations have been developed. Also, there are several modern generic versions of current medications, but none have enough data to support their mass usage. The World Health Organization (WHO) suggested artemisinin combination therapy (ACT) as an initial therapy for uncomplicated malaria patients. ACT combines rapidly acting artemisinin derivatives with a longer-acting (slowly eliminated) antimalarial drug. The artemisinin derivative rapidly clears parasites from circulation. The longer-acting antimalarial drug with higher elimination half-lives remove the remaining parasites and provide a period of post-treatment prevention [2]. However, ACT is associated with a nearly 50% cure failure rate in some areas in Southeast Asia [3]. ACT-resistant Plasmodium falciparum has emerged, and its area of infection is expanding [4,5]. The WHO malaria eradication program includes discovering new compounds with antiparasitic action and a high therapeutic index with minimal toxicity [6]. Therefore, to overcome ACT and other drug-resistant P. falciparum, there is an urgent need to develop new antimalarial drugs structurally distinct from the available drugs and target different metabolic pathways [7].
We discovered a new antimalarial drug from synthetic endoperoxide compounds, 1,2,6,7- tetraoxaspiro[7.11]nonadecane (N-89) [8]. N-89 exhibited strong antimalarial activity in vitro [8–11] and was used as a potential candidate to treat childhood malaria in ointments. This study explored the different formulations that can be applied to skin and area sizes to maintain an effective N-89 plasma concentration for a prolonged time [12]. Additionally, a combination therapy involving N-89 with mefloquine or pyrimethamine was effective against P. berghei-infected mice [13]. To determine the suitable antimalarial candidates using N-89 structure-based derivatives, 6-(1,2,6,7-tetraoxaspiro[7.11]nonadec-4-yl) hexan-1-ol (N-251), having similar antimalarial activity as N-89, was selected. The antimalarial activity and cure effects of N-251 without recurrence and safety test results have been previously reported [11,14]. Additionally, an analytical detection method was developed for N-251 [15,16]. During the development of N-251 as a new antimalarial drug, issues like low yields following multiple synthetic steps have been reported; therefore, different synthetic preparation methods for N-251 are being developed [17].
The exploration of antimalarial mechanisms of N-89 demonstrated that P. falciparum endoplasmic reticulum resident calcium-binding protein (PfERC) could be a possible target [9,18], with inhibition activities during the third stage of parasite development inside red blood cells [10]. Although the functions of PfERC have not been elucidated, it may play an essential role in the division, recruitment, and protein export of malaria parasites [19–21].
A pharmacokinetic study is required to determine the suitable treatment schedule for N-89, a medication that can be of practical application. This report aimed to conduct a pharmacokinetic (PK) study for N-89 and characterize the antimalarial activity and cure effects of oral N-89 based on the PK data for the first time. We also tested the efficacy of N-89 using different administration routes.
Materials and Methods
Materials
N-89 (Fig. 1, inset) was produced in our laboratory as follows. First, methoxymethylenecy-clododecane and cyclododecanone were prepared by ozonolysis of a vinyl ether in the presence of hydrogen peroxide in diethyl ether. Next, (cyclododecylidene)bishydroperoxide was produced by combining methoxymethylenecyclododecane and cyclododecanone in the presence of peroxide and ozone in acidic conditions. N-89 was produced from (cyclododecylidene)bishydroperoxide and CsOH·H2O in DMF [8]. Olive oil was procured from Wako (Osaka, Japan), and Chremophor EL was purchased from Sigma (St. Louis, MO, USA). All other chemicals and reagents were of analytical grade.
Mice and parasites
Institute of Cancer Research (ICR) male mice (The Jackson Laboratory, Kanagawa, Japan) and P. berghei (NK65 strain) parasites were maintained in the laboratory. P. berghei (NK65 strain) infected ICR mice died from parasite infection within one week. The experiment collected blood from a donor mouse previously infected with P. berghei when the parasitemia reached 15%. The parasitized blood was intravenously (iv) injected into mice, and P. berghei-infected mice were developed. We used ICR mice and P. berghei (NK65 strain) to study the compounds’ antimalarial activity and cure effects. The mice were reared in a 12 h light/dark cycle at room temperature (23±2°C) with free access to water and food (MF, Oriental Co. Ltd., Tokyo, Japan). The Institutional Animal Care and Use Committee of Okayama University granted consent for this study (approval number: OKU-2017199), which was carried out per the university’s guidelines.
Pharmacokinetic analysis of oral N-89
To evaluate the pharmacokinetics of N-89 in mice, a specific and sensitive liquid chromatography/tandem mass spectrometric (LC/MS/MS) method was used [15]. N-89 was administered at a single dose of 75 mg/kg orally (po) as a solution in olive oil or 30 mg/kg intravenous administration as a solution in 10% ethanol, 10% Cremophore EL, and 80% saline (1:1:8 [v:v:v]). The blood samples were collected at 0.25, 0.5, 0.75, 1, 2, 3, 4, 5, 8, and 10 h post-treatment. As it is challenging to collect multiple blood samples from a single mouse, a blood sample was collected once from a mouse at a given time, and 5 mice were used each time. Fifty mice were used for blood sample collection. The collected blood samples were centrifuged for 10 min at 945 g at 4°C. N-89 was isolated from the plasma samples using acetonitrile as a solvent, and further steps were performed as described by Okada [16]. The plasma concentration of N-89 was detected by LC/MS/MS system (LC; Agilent 1200, Agilent Technologies, Tokyo, Japan, MS/MS; API4000, AB SCIEX, Tokyo, Japan). The TSKgel Super-octyl column (Tosoh Co., Tokyo, Japan) was used to analyze a sample of 10 μl Acetonitrile: 10 mM ammonium acetate (8:2) was used as a mobile phase and supplied at 0.2 ml/min. The ESI interface in the positive MRM mode was used for N-89 measurement. The used parameters were adjusted as described by Imada [15]. N-89 parent ions (m/z, 273) and a monitoring ion 183 were detected. Multiple standard curves were created for the 5–1,000 ng/ml concentration ranges, with a correlation coefficient above 0.994.
PK parameters of N-89 were calculated using a macro program, MOMENT (EXCEL) [22,23], running on Microsoft Excel by model-independent analysis. The highest detected plasma concentration of oral N-89 (Cmax) and the time required to reach the Cmax (Tmax) values were obtained from the experimental data. The time required to reach half of Cmax is t1/2. The area under the plasma concentration-time curve (AUC) and mean residence time were estimated from the single time course detected with mean values. All the data were expressed as the mean value (n=5). Finally, the bioavailability (F) was calculated according to the formula. F (%)=100×AUCpo×Doseiv/AUCiv×Dosepo.
Antimalarial activity of N-89 using different administration routes (4-day suppressive test)
The antimalarial activity of N-89 using different treatment routes was examined using an in vivo Peters’ 4-day suppressive test as described previously [24]. In the study, each healthy mouse was infected with P. berghei (NK65 strain) parasites by iv infusion of 1×105 infected erythrocytes. N-89 was administered to mice at doses of 0, 10, 20, 40, and 80 mg/day for 4 days (days 0, 1, 2, and 3), either po (as a solution in olive oil), or iv (as a solution in 10% ethanol, 10% Chremophore EL, and 80% saline (10:10:80 (v:v:v)). N-89 was administered subcutaneously (sq) at doses of 0, 1, 2, 4, and 8 mg/day for 4 days (as the same solution of iv treatment). N-89 administration on day zero was performed after 2 h from parasite inoculation. The control group received the vehicles only. Five mice were used in each group. The parasitemia was determined on day 4 by performing thin blood films.
Antimalarial activity following repeated oral dosing (Cure effect)
The mice were tested by iv infusion of 1×106 infected erythrocytes (day 0). Mice with more than 0.5% parasitemia were administered N-89 orally at 50 or 75 mg/kg thrice daily for three days (days 2, 3, and 4) based on the PK data. The changes in parasitemia and survival of mice were observed for 30 days.
Statistical analysis
The results were tabulated and analyzed using Microsoft Excel 2016. Percent parasitemia was calculated by dividing the number of infected erythrocytes by the total number of erythrocytes indexed and multiplied by 100. The results were expressed as the percent reduction of parasitemia compared to untreated control. ED50 and ED90 values (effective dose leading to the death of 50% and 90% parasites) were obtained by sigmoidal dose-response curve analysis. The data were expressed as the mean±standard deviation (SD).
Results
Pharmacokinetic analysis of N-89
The N-89 plasma concentration after a single po and iv administration is shown in Table 1 and Fig. 1. For iv administration, the plasma concentration of N-89 declined time-dependently (Fig. 1). The concentration of N-89 was 18.32 μg/ml at 0.25 h and could not be detected at 5 h, demonstrating its very short residence time in the blood and rapid metabolism or circulation in the body. The t1/2 was 1.5 h and AUC0→last and AUC0→∞ were 13.57 and 13.59 μg·h/ml, respectively. After oral N-89 treatment, the Cmax was 1.59 μg/ml at 0.75 h and t1/2 was 0.97 h. A plasma concentration of 8.1 ng/ml was detected at 8 h but could not be detected at 10 h (the detection limit of the LC/MS/MS system was 5 ng/ml). The AUC0→ last was 2.37 μg·h/ml, and AUC0→∞ was 2.38 μg·h/ml. Finally, F was calculated as 7.01%, indicating a low oral bioavailability of N-89 in ICR mice. The dotted line in Fig. 1 indicated the EC50 value of P. falciparum (6.8 ng/ml) [8], and a single oral N-89 dose of 75 mg/kg remained an effective concentration for inhibition of 50% of parasites for 8 h. The plasma level of oral N-89 was between 10 and 1.59 μg/ml within 8 h and did not accumulate in the mice.
Antimalarial activity of N-89 using different administration routes (4-day suppressive test)
The N-89 antimalarial activity was examined by different routes, specifically, po, iv, and sq. The ED50 and ED90 values are indicated in Table 2. The ED50 values were 20.5, 14.5, and 2.5 mg/kg, respectively. Low doses of sq N-89 showed high antimalarial activity compared to other treatment routes. However, after stopping the sq N-89 treatment, parasitemia increased anew, and all N-89 treated mice died on day 8. All control mice died on day 7 (mean survival was 6.8 days).
Antimalarial activity following repeated oral dosing (Cure effect)
A cure effect means that the P. berghei-infected mice had no parasites for up to one month following N-89 treatment. We evaluated the cure effect and changes in parasitemia following 75 mg/kg N-89 treatment based on the plasma concentrations of the oral data (8.1 ng/ml of N-89 was detected at 8 h). We fixed the treatment schedule thrice daily for 3 consecutive days using 0.5% parasitemia as a malaria patient model (on day 2). Oral administration of N-89 caused a decrease in parasitemia to day 3, and no parasites were observed in the blood from day 5 to 30 without parasite recurrence (Fig. 2). Parasitemia reduction below the level of detection was achieved on days 4–5 (i.e., 2–3 days after oral dosing).
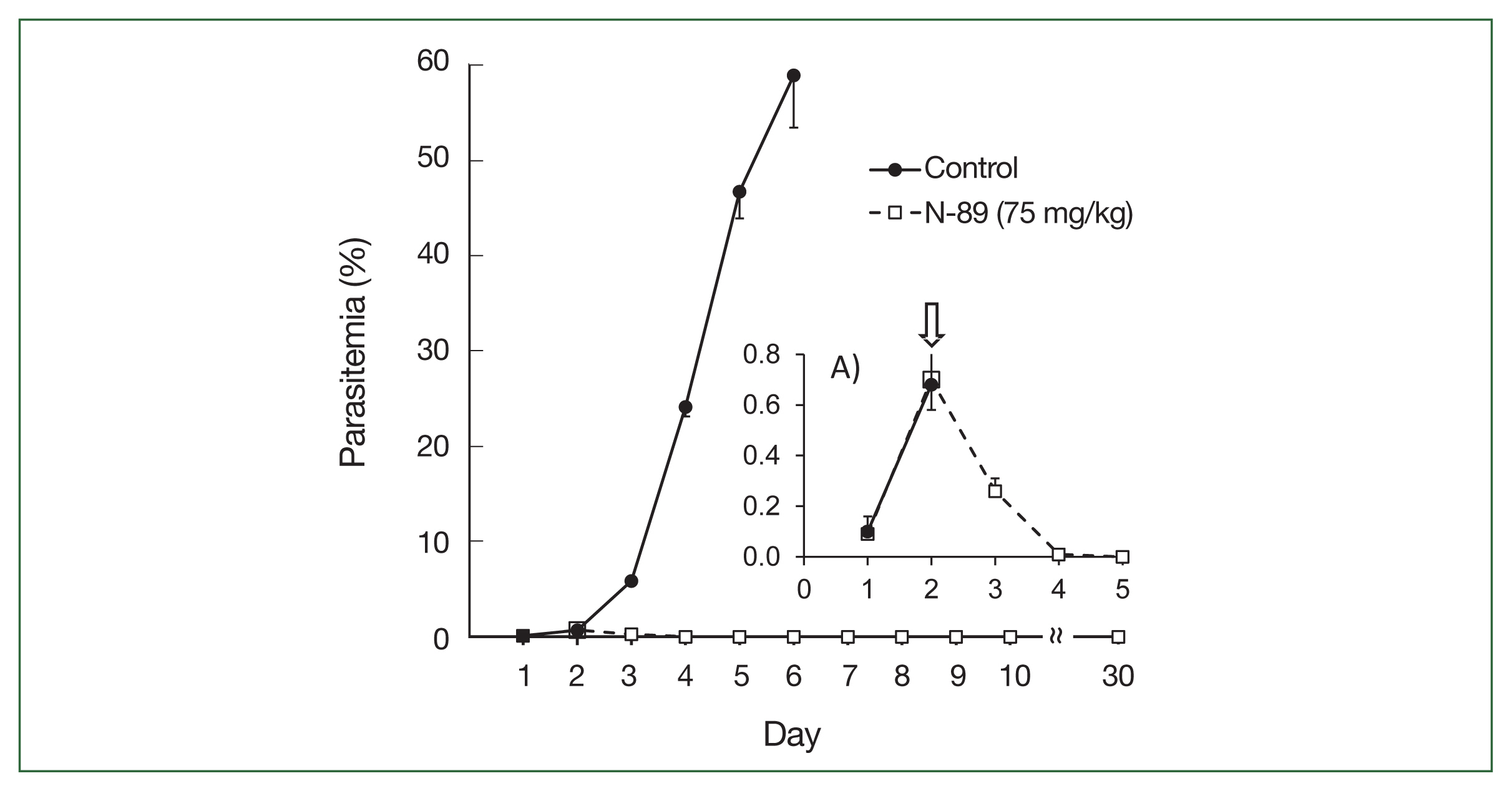
Antimalarial activity of N-89 (po, 75 mg/kg). Mice with more than 0.5% parasitemia, 75 mg/kg of N-89 administered 3 times daily for 3 days. The data are presented as the mean, with a bar indicating the SD value. A) Inset details the parasitemia from day 1 to 5. An arrow indicated the start time of the N-89 treatment.
The upper section of Table 3 indicates a detailed parasitemia with SD values from day 1 to 6 to evaluate the antimalarial activity during or after N-89 treatment. One mouse was cured with no parasites in the bloodstream and 4 mice had low parasitemia (below 0.015%) on day 4. All mice were cured on day 5. The survival times were determined by observing mice for 30 days. N-89 treated mice survived for more than 30 days while the mean survival day of the control mice was 5.6 (Table 3). These results reflected that the PK data of oral N-89, and 75 mg/kg of N-89 was sufficient for parasite elimination. Therefore, we studied the antimalarial activity and cure effect using a lower dose of N-89 (50 mg/kg) with the same experimental conditions.
Oral administration of a low dose of the N-89 treated group showed a decrease in parasitemia on day 3, and no parasites were observed in the blood from day 6 to 30 without parasite recurrence (Fig. 3).
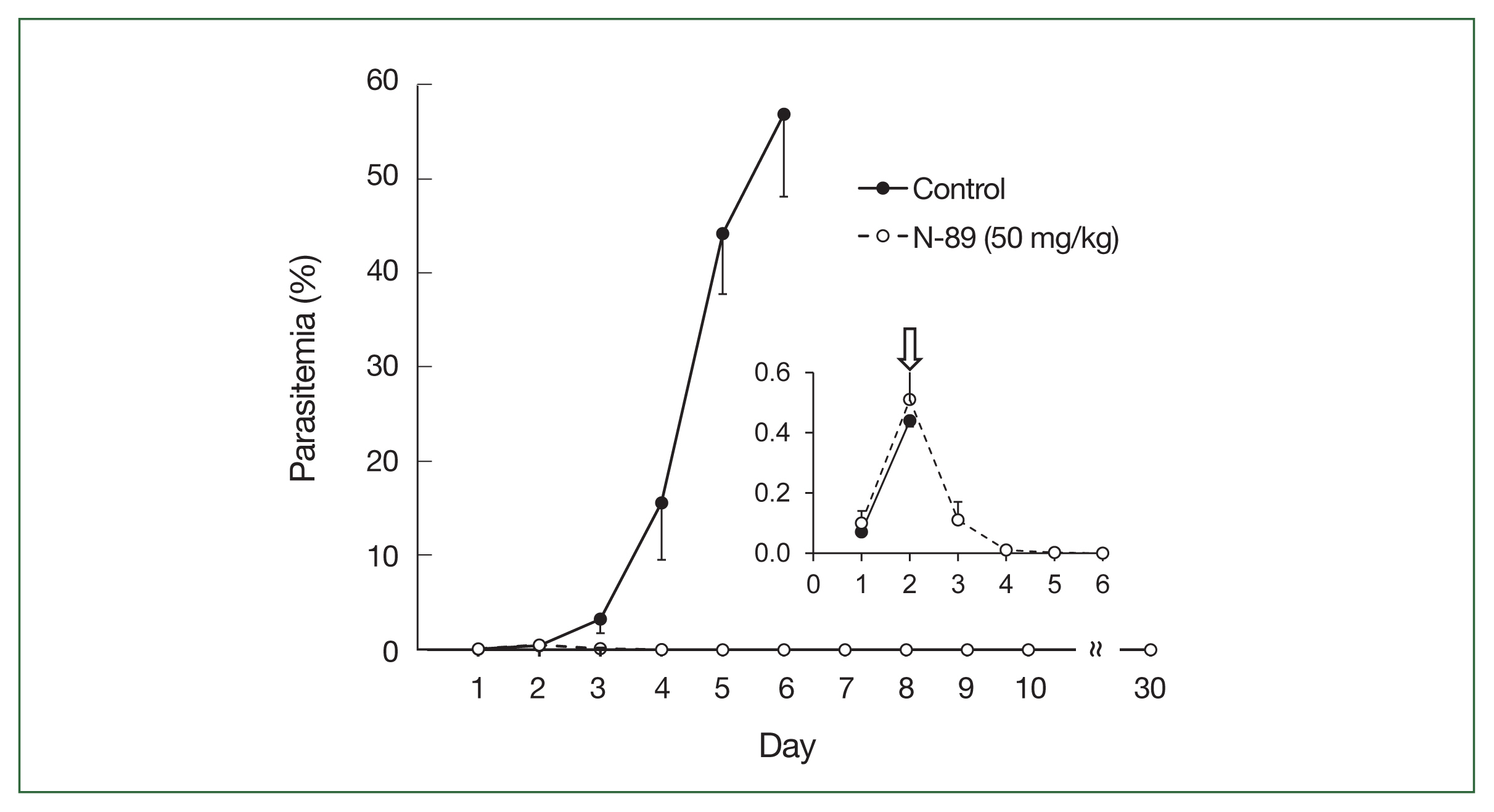
Antimalarial activity of N-89 (po, 50 mg/kg). Mice with more than 0.5% parasitemia, 50 mg/kg of N-89 administered thrice daily for 3 days. The data are presented as the mean, with a bar indicating the SD value. The inset details the parasitemia from day 1 to 6. An arrow indicated the start time of the N-89 treatment.
The lower section of Table 3 indicates a detailed parasitemia with SD values from day 1 to 6. Four mice were cured, one mouse showed 0.01% parasitemia on day 5, and all were cured on day 6. N-89 treated mice survived for more than 30 days, while the mean survival day of the control mice was 6.2 (Table 3). This data indicated that 50 mg/kg of N-89 exhibited a complete cure effect for the malaria parasite in mice.
Discussion
Oral medication is the most popular drug administration route. Current estimates indicate that oral medication represents about 90% of the global market share of all pharmaceutical preparations designed for human use [25]. The current study aimed to accelerate antimalarial drug development efforts by investigating the potential use of the N-89 drug via different application routes to target malaria infection. Pharmacokinetics in animal studies is required to develop N-89, and the appropriate administration schedule of effective N-89 can be confirmed by detecting the PK parameters in this study. Its antimalarial effect is comparable to that of artemisinin, and its toxicity against P. falciparum is 350-fold more than that against mouse mammary tumor FM3A cells in vitro [11], indicating its selective toxicity against malaria parasites.
This study was the first to report the PK data of N-89. We evaluated the antimalarial activity using a mice model for malaria patients based on the plasma concentration of oral N-89. PK analysis of N-89 indicated that the plasma concentration of N-89 was 8.1 ng/ml and was maintained for 8 h (Fig. 1). In a previous report [8], the N-89 EC50 value against P. falciparum was 6.8 ng/ml; therefore, a plasma concentration of 8.1 ng/ml could inhibit parasites in mice. The plasma level of oral N-89 within 8 h did not accumulate in mice. The sigmoidal pattern of plasma concentration of oral N-89 indicated that the maximum concentration was 1.59±1.11 μg/mL at 0.75 h and then decreased to 8.1 ng/ml after 8 h as shown in Fig. 1. The results suggested that N-89 showed antimalarial activity without accumulation effects with multiple oral administrations in the body. The t1/2 of oral N-89 was 0.97 h (Table 1) and multiple administrations would be needed to maintain an effective plasma level of N-89. Therefore, in this study, we chose a 3-daily dosing plan of oral N-89 (50 mg/kg) for a cure effect. The oral N-89 treated group (50 mg/kg, thrice daily for 3 days) caused parasite killing, and mice were cured (Fig. 3). The F of N-89 was calculated for the first time, and it was 7.01%. The F of N-89 would be difficult to further drug development study, but a single oral N-89 compound had a complete cure effect without recurrence. To develop the next stage, we improved the detection methods and re-evaluated the PK analysis with a detailed metabolic analysis of N-89. Endoperoxide structure containing artemisinin and its derivatives reported PK data and its bioavailability showed a broad range from 1.60–26.1% using rats. [26]. There are challenges in the direct comparison of F value and needs further studies to understand detailed PK parameters using an improved N-89 detection method.
Previously, we studied the effects of an ointment of N-89. The transdermal treatment with N-89 was an effective and safe alternative route for treating malaria. The cure effect of N-89 in mice was tested at 60 mg/kg twice daily for 4 days at 0.2% parasitemia. Parasites disappeared post-treatment from day 7 in N-89 treated groups without parasite recurrence or dermal irritation [12].
Klayman et al. [27] developed a gel containing artelinic acid that was applied topically (twice/day×3 days) post-infection (dose 270 mg/kg). They prevented the death of mouse-infected P. berghei. Also, they reported that the improved formulation containing artemether instead of artelinic acid in the same experimental conditions was efficient at a lower dose of 60 mg/kg [28].
The current study examined the antimalarial activity of N-89 using a 4-day suppressive test by different routes; po, iv, and sq. Specifically, sq N-89 has antimalarial activity with a low dose of N-89. Still, sq treatment needs the support of medical staff with a disposable syringe during malaria treatment, and we did not perform further studies using sq N-89. Oral treatment is a preferable route of administration in non-complicated adult malarial patients or visitors to endemic areas because of convenience and no need for hospitalization or special techniques. Thus, we evaluated the detailed antimalarial activity and cure effect of oral N-89.
In conclusion, the data presented in this study showed the pharmacokinetics of N-89 and its complete cure effect for the first time. For the clinical application of oral N-89 in treating malaria patients, a suitable administration schedule and its metabolic pathways of N-89 should be explored.
Acknowledgments
We thank Tomoko Tanaka and Kazuaki Okada (Okayama University) for performing experiments and discussing the results. This study was supported in part by a grant from the Program of the Japan Initiative for Global Research Network on Infectious Diseases (J-GRID, JP22wm0125004) from the Ministry of Education, Culture, Sports, Science, and Technology in Japan (MEXT) and the Japan Agency for Medical Research and Development (AMED).
Notes
Author contributions
Conceptualization: Aly N, Rashed GA, Kim HS
Formal analysis: Yu HS
Funding acquisition: Miyoshi SI, Kim HS
Investigation: Aly N, Matsumori H, Dinh TQ
Methodology: Matsumori H, Dinh TQ
Project administration: Kim HS
Resources: Miyoshi SI, Kim HS
Software: Yu HS, Cao DT
Supervision: Sato A, Kubota T, Kurosaki Y, Kim HS
Validation: Aly N, Sato A, Miyoshi SI, Chang KY, Yu HS, Kubota T, Kurosaki Y, Cao DT, Kim HS
Visualization: Miyoshi SI, Kubota T, Kurosaki Y
Writing – original draft: Aly N
Writing – review & editing: Chang KY, Cao DT, Rashed GA, Kim HS
The authors have no conflicts of interest.