In vitro anti-Trypanosoma cruzi activity of methanolic extract of Bidens pilosa and identification of active compounds by gas chromatography-mass spectrometry analysis
Article information
Abstract
Chagas disease, caused by Trypanosoma cruzi parasite, is a significant but neglected tropical public health issue in Latin America due to the diversity of its genotypes and pathogenic profiles. This complexity is compounded by the adverse effects of current treatments, underscoring the need for new therapeutic options that employ medicinal plant extracts without negative side effects. Our research aimed to evaluate the trypanocidal activity of Bidens pilosa fractions against epimastigote and trypomastigote stages of T. cruzi, specifically targeting the Brener and Nuevo León strains—the latter isolated from Triatoma gerstaeckeri in General Terán, Nuevo León, México. We processed the plant’s aerial parts (stems, leaves, and flowers) to obtain a methanolic extract (Bp-mOH) and fractions with varying solvent polarities. These preparations inhibited more than 90% of growth at concentrations as low as 800 μg/ml for both parasite stages. The median lethal concentration (LC50) values for the Bp-mOH extract and its fractions were below 500 μg/ml. Tests for cytotoxicity using Artemia salina and Vero cells and hemolytic activity assays for the extract and its fractions yielded negative results. The methanol fraction (BPFC3MOH1) exhibited superior inhibitory activity. Its functional groups, identified as phenols, enols, alkaloids, carbohydrates, and proteins, include compounds such as 2-hydroxy-3-methylbenzaldehyde (50.9%), pentadecyl prop-2-enoate (22.1%), and linalool (15.4%). Eight compounds were identified, with a match confirmed by the National Institute of Standards and Technology (NIST-MS) software through mass spectrometry analysis.
Introduction
According to the World Health Organization, neglected tropical diseases (NTDs) disproportionately afflict individuals of lower socioeconomic status, presenting significant health challenges to millions globally and perpetuating cycles of poverty and illness. Among these NTDs, American trypanosomiasis, better known as Chagas disease, is a notable concern. This disease, caused by the flagellate protozoa Trypanosoma cruzi [1], represents a significant socioeconomic burden, particularly in Latin America, encompassing South and Central America and México. There is an urgent need for improved medical care to enhance the quality of life for the growing patient population, especially in the chronic phase characterized by severe complications such as megacolon, megaesophagus, and cardiomegaly. Current treatments for Chagas disease show limited efficacy in the chronic stage, typically diagnosed in adulthood via serological tests. Novel alternatives, including natural product extracts, are being explored to address these limitations [2].
The vast diversity of medicinal plants in México offers potential health benefits. Bidens pilosa—commonly known as hairy beggarticks—is native to North America and prevalent in México. It has been recognized for its antimicrobial properties and specific antiprotozoal effects against various parasitic genera [3]. Various parts of B. pilosa have exhibited activity against parasites such as Giardia duodenalis, Plasmodium falciparum, Leishmania amazonensis, and T. brucei rhodesiense [4,5].
This study aims to evaluate the efficacy of methanolic extracts from B. pilosa against the epimastigote and trypomastigote stages of T. cruzi. It also seeks to biochemically identify the most potent trypanocidal fraction using mass spectrometry. Additionally, the cytotoxic and hemolytic effects of B. pilosa extracts were assessed to ensure safety for subsequent trials in murine models.
Materials and Methods
Ethical statement
Artemia salina is not considered a threat to the environment and is not listed as an endangered species. It does not feature in any European or international catalog of protected species, including the Convention on International Trade in Endangered Species of Wild Fauna and Flora (CITES) list (www.cites.org) or the International Union for the Conservation of Nature Red List.
Plant material and parasite strains
All materials were harvested in June 2020 within Monterrey [25°36′19.9″N 100°15′16.7″W], San Nicolás de los Garza [25°43′33.9″N 100°18′37.3″W], and Guadalupe [25°39′25.4″N 100° 15′14.7″W], located in Nuevo León, México. Taxonomic identification of the plant was performed at the Herbarium of the Botany Laboratory, UANL, where it was cataloged under the number 030599. We used 2 T. cruzi strains: the Brener and NL strains (isolated from Triatoma gerstaeckeri collected in Nuevo León, México), both provided by the Laboratory of Molecular and Experimental Pathology (UANL).
Plant extraction
The aerial parts of B. pilosa were dried at ambient temperature. Subsequently, a 25 g sample was pulverized to a fine powder using a mortar. This powder was subjected to extraction in a Soxhlet apparatus using 500 ml of absolute methanol (TEDIA, Fairfield, OH, USA), with indirect heating facilitated by a magnetic stirrer set at 60 V (C-MAG HS 10, Carl Roth, Karlsruhe, Germany) for 48 h [3,5]. The methanolic extract was then filtered using Whatman filter paper No. 1 and the filtrate was concentrated at 45°C under vacuum using a rotary evaporator (Yamato Digital, RE301, Santa Clara, CA, USA). The residual solvent was removed in a vacuum desiccator at room temperature, and the dry extract was weighed to determine the yield percentage [6]. The resulting extract, termed Bp-mOH, was stored in dark glass vessels, wrapped in aluminum foil to protect from light, and kept at 4°C for future use.
Subsequently, fractions of Bp-mOH were derived by sequentially partitioning the solid extract with solvents of varying polarities. Silica gel column chromatography was employed to separate the extract, first eluting with chloroform. Further fractionation was achieved using a mixture of chloroform and ethyl acetate in a 1:1 ratio (the fraction was designated as BPFC1CL1); a 1:1 mixture of ethyl acetate and methanol (fraction BPFC2AE1); and a 1:1 mixture of chloroform and methanol (fraction BPFC3MOH1). Yields for each partition and fractionation step were calculated using the previously described methods [7].
Phytochemical analysis
Various phytochemical qualitative techniques were performed on the extract and fractions of B. pilosa using several standard methods: Molisch’s test (for carbohydrates), Millon’s test (for amino acids), Shinoda’s test (for flavonoids), Biuret’s test (for proteins), the ferric chloride test (for phenols and enols), Foam’s test (for saponins), Salkowski’s test (for cholesterol and sterols), and Wagner’s test (for alkaloids) [8].
Parasites and maintenance conditions
Epimastigote forms were cultured in Liver Infusion Tryptose medium (ThermoFisher Scientific, MA, USA) supplemented with 10% fetal bovine serum until they reached the exponential growth phase, with a cell density of 1×106 epimastigotes/ml [9]. Metacyclic trypomastigotes were induced using the technique described by Contreras et al. [10]. Nifurtimox (Sigma-Aldrich, St. Louis, MO, USA) at a concentration of 100 μg/ml was used as a positive control and dimethyl sulfoxide (DMSO 1%) served as the solvent for the plant extract [11].
Anti-Trypanosoma cruzi activity
The anti-T. cruzi activities of the Bp-mOH plant extract and its fractions were conducted in vitro using epimastigotes and trypomastigotes obtained from the T. cruzi cultures in 96-well plates (1×106 parasites/well). Different concentrations (300–3,000 μg/ml) of the plant extract and fractions, dissolved in 1% DMSO, were used in triplicate. The susceptibility to the extract and its fractions was assessed using the enzymatic micro-method involving MTT (3-(4,5-dimethylthiazol-2-yl)-2,5-diphenyl tetrazolium bromide) [11].
Parasites in the exponential growth phase at a 1×10 6 parasites/ml concentration were subjected to this assay. The effect of the extract/fractions on the parasites was determined by measuring mitochondrial dehydrogenase activity, achieved by adding 10 μl/well of MTT and phenazine methosulfate (PMS, 0.2 mg/ml of MTT solution) [11]. Absorbance was read at 570 nm on a microplate reader, ASYS UVM-340 spectrophotometer (Biochrom, Cambridge, UK), and the data were analyzed to calculate the lethal concentration of 50% (LC50) [11].
Analysis via fractionation and gas chromatograph-mass spectrometry (GC-MS)
A 22×300 mm glass chromatographic column was prepared with 20 g of silica gel (Sigma-Aldrich). Subsequently, 50 mg of the extract to be fractionated was added. The column was eluted with 10 ml of solvents with increasing polarity, and 5 ml fractions were collected. The collected fractions were evaporated using a rotary evaporator, and those with similar chromatographic profiles were combined to create 3 composite fractions; BPFC1CL1, BPFC2AE1, and BPFC3MOH1 [7].
Volume samples were adjusted to 1 ml for qualitative analysis. Initially, they were spotted on 5 cm×10 cm thin-layer chromatography (TLC) plates. TLC was performed using various solvent systems, including chloroform, ethyl acetate, methanol, chloroform-ethyl acetate 1:1, ethyl acetate-methanol 1:1, and chloroform-methanol 1:1. Afterward, the chromatoplates were air-dried for 24 h, and fluorescent bands were visualized under short and long wavelength UV light (254 nm and 365 nm) using a UV transilluminator. Compounds that did not absorb UV light required a developing agent, and the plates were sprayed with sulfuric acid (H2SO4) and documented accordingly [12]. The retention factor (Rf) was calculated as previously described [12].
Compound identification was carried out using a gas chromatography system (7890A, Agilent Technologies, CA, USA) coupled with a single-quadrupole mass spectrometer (5975C VL MSD, Agilent Technologies) (GC-MS). Chromatographic separation was achieved on an HP-5MS fused-silica capillary column, following the manufacturer’s instructions. The spectrum of the unknown components was compared with the standards in the National Institute of Standards and Technology (NIST) library database [13].
Cytotoxicity assay
Brine shrimp eggs (Artemia salina, INVE, Aquaculture, NV, USA) were hatched in a 10 L glass aquarium filled with artificial seawater (Kent Marine, GA, USA) at a salinity of 38 ppt, following the procedure described by Mclaughlin [14]. Various concentrations (3,000, 2,500, 2,000, 1,500, 1,100, 950, 800, 650, and 300 μg/ml) were evaluated at a 25–30°C temperature range for 24 h with continuous illumination. Potassium dichromate (K2Cr2O7) at a concentration of 100 μg/ml was the positive control, while 1% DMSO was the negative control. Following 24 h of exposure, the count of live and dead larvae was recorded to assess statistical significance using Probit analysis in SPSS for determining the LC50 [14].
Furthermore, due to the initial mild to moderate toxicity results, a cytotoxic assay was conducted. In brief, the toxicities of the plant extract and fractions (Bp m-OH) were assessed in Vero cells (ATCC, CCL-81) using the MTT assay as previously described [11]. Vero cells were suspended in Gibco Roswell Park Memorial Institute (RPMI 1640) medium (Thermo Fisher Scientific) supplemented with 10% fetal bovine serum in a 5% CO2 atmosphere at 37°C. Vero cells were adjusted to a concentration of 2.2×105 cells in 96-well microplates and incubated 24 h before treatment. Subsequently, cells were exposed to different concentrations of extracts/fractions (ranging from 300 to 3,000 μg/ml) for the next 24 h under the same conditions mentioned above. Afterward, 20 μl of MTT reagent (5 mg/ml) was added to each well, followed by an additional 1 h incubation. Next, 100 μl of DMSO was added, and the optical density was measured at 540 nm using an ELISA Variouskan LUX multimode microplate reader (Thermo Fisher Scientific). In this assay, the negative control consisted of RPMI medium with Vero cells and DMSO. Additionally, cell viability was assessed microscopically for the presence or absence of cytopathic effects. Each trial of extract/fraction was performed in triplicate. Cytotoxic activity was expressed as the concentration required to reduce cell viability by half (CC50) after 24 h of treatment. The CC50 value and the standard error mean (SEM) were calculated using a non-linear regression curve in SPSS, following Meyer et al.’s toxicity criteria [15]. Furthermore, toxicity was related to the trypanocide activity (the concentration required for 50% growth inhibition, IC50, and LC50) by determining the selectivity index (SI) [16]. The extracts/fractions against T. cruzi were categorized as non-selective (SI<2), moderately selective (SI of >2 and <3), and highly selective (SI>3) [16].
Hemolysis assay
Hemolytic activity was determined by assessing the hemolysis of a suspension of human erythrocytes from healthy O Rh (+) donors. Human blood was collected using venipuncture into a purple tube containing EDTA as an anticoagulant. The experiment followed the method outlined by Elizondo-Luevano et al. [17,18]. The red blood cell suspension was incubated with various concentrations from 300 to 3,000 μg/ml of the extracts/fractions in pre-prepared aliquots in 2 ml microcentrifuge tubes for 30 min at 37°C while being protected from light. The absorbance values of the supernatants were measured at 405/540 nm using a microplate reader, ASYS UVM-340 [19]. The negative control consisted of untreated erythrocytes, and sterile distilled water was used as the positive control to induce osmotic hemolysis. The recorded value represented each treatment’s absorbance. The percent hemolysis was calculated as previously described [19].
Statistical analysis
The results were expressed as the mean±SD. One-way analysis of variance (ANOVA) was employed to assess the statistical significance of the extracts/fractions at various concentrations, and multiple comparisons of means were conducted using the Tukey test (post-Hoc) (P≤0.05). Probit analysis with a 95% confidence interval was also utilized to determine the IC50. All analyses were conducted in triplicate and calculated using SPSS software.
Results
Plant material and phytochemical analysis
The yield percentages for Bp-mOH crude extract and the fractions BPFC1CL1, BPFC2AE1, and BPFC3MOH1 were 26.2%, 1.4%, 5.8%, and 12.9%, respectively. The highest yield of the crude extract was obtained from the methanolic fraction of the aerial parts of B. pilosa. Our results indicated that the fraction exhibiting the most potent activity against the parasite was BPFC3MOH1. Basic phytochemical analysis revealed the presence of phenolic compounds, alkaloids, carbohydrates, and proteins, among the most prominent components.
Characterization of extracts
Rf values for the methanolic extract from B. pilosa were observed via TLC for the collective fractions BPFC1CL1, BPFC2AE1, and BPFC3MOH1. Chromatographs under visible light and UV spectrum (254 nm) are depicted (Fig. 1).
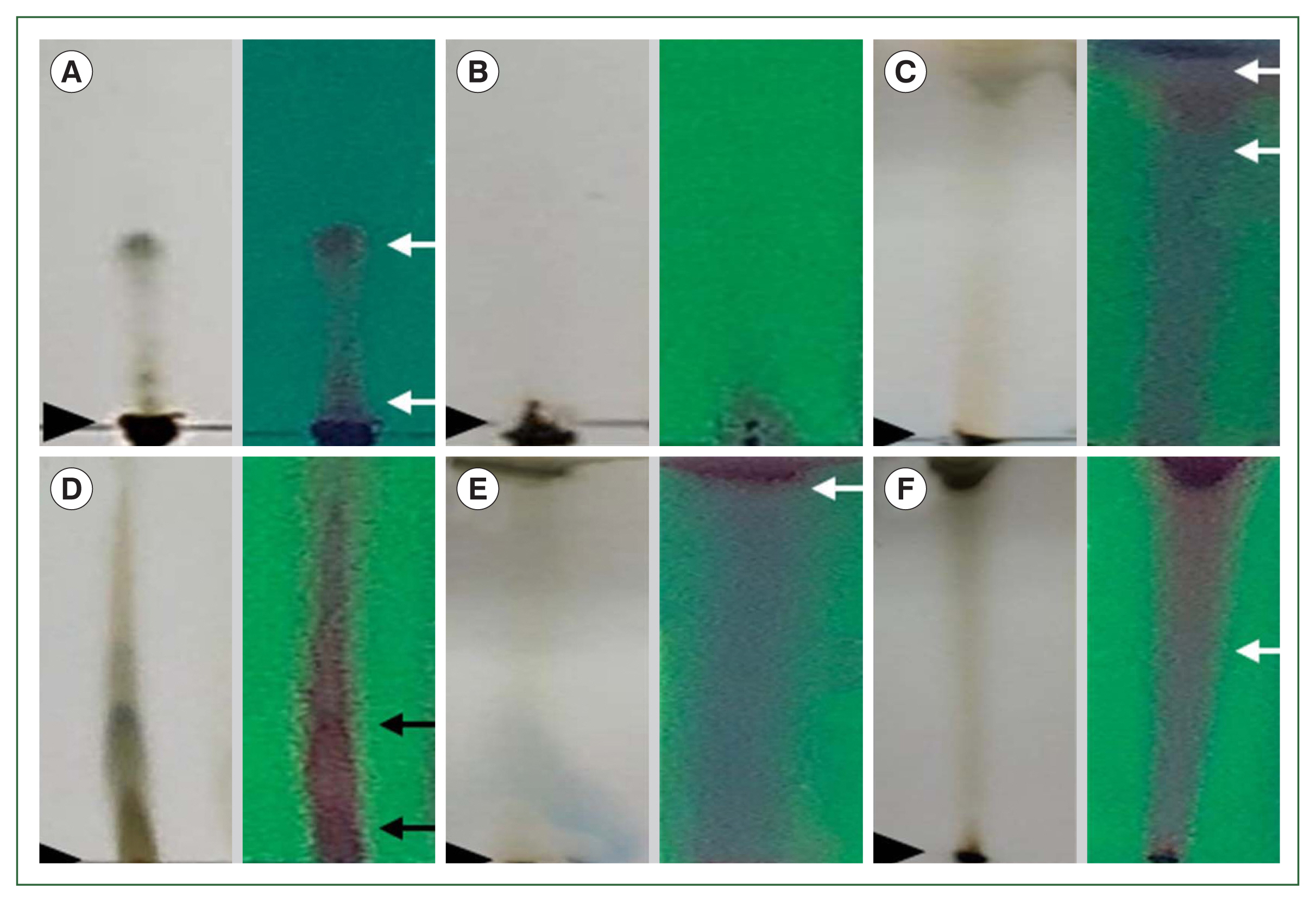
Representative results of thin layer chromatography (TLC) analysis with visible light at left and UV spectrum (254 nm) at right. Origin is indicated with arrow heads and Rf with 2 arrows. (A) Chromatographs with chloroform eluent show 2 spots (Rf of 0.07 and 0.17). (B) Ethyl acetate eluent no spot was observed. (C) Methanol eluent the Rf was of 0.82 and 0.88. (D) Chloroform-ethyl acetate 1:1 eluent 2 spots were observed (Rf of 0.04 and 0.15). (E) Ethyl acetate-methanol 1:1 eluent and one spot (Rf= 0.88) was observed. (F) Chloroform-methanol 1:1 eluent the chromatograph shows 1 spot (Rf=0.88), all of which were from methanolic B. pilosa extract.
The BPFC3MOH1 fraction was characterized using GC-MS (Fig. 2A, B). Among the signals with the highest intensities, the retention times were attributed to the following compounds: linalool (2.3 min), ethyl 2-nonynoate (2.4 min), 2-hydroxy-3-methylbenzaldehyde (2.6 min), 2,6-nonadienal, 3,7-dimethylnona-2,6-dienal (2.8 min), pentadecyl acrylate (5.2 min), hydroquinone (13.6 min), 2-hydroxy-5-methyl benzaldehyde (16.6 min), and dodecyl acrylate (21.4 min). All compound data were matched using NIST-MS software. Table 1 and Fig. 2 present various molecules identified from the BPFC3MOH1 fraction and additional characteristics such as synonyms, molecular formula, chemical composition, and their respective percentages in the sample. Notably, 2-hydroxy-3-methylbenzaldehyde accounted for the majority, with a percentage of abundance at 50.9%, followed by pentadecyl prop-2-enoate with 22.1%. Details of other compounds can be found in Table 1. Further information on the mass spectrum, chemical structure, and abundance of the compounds is provided in Fig. 2.
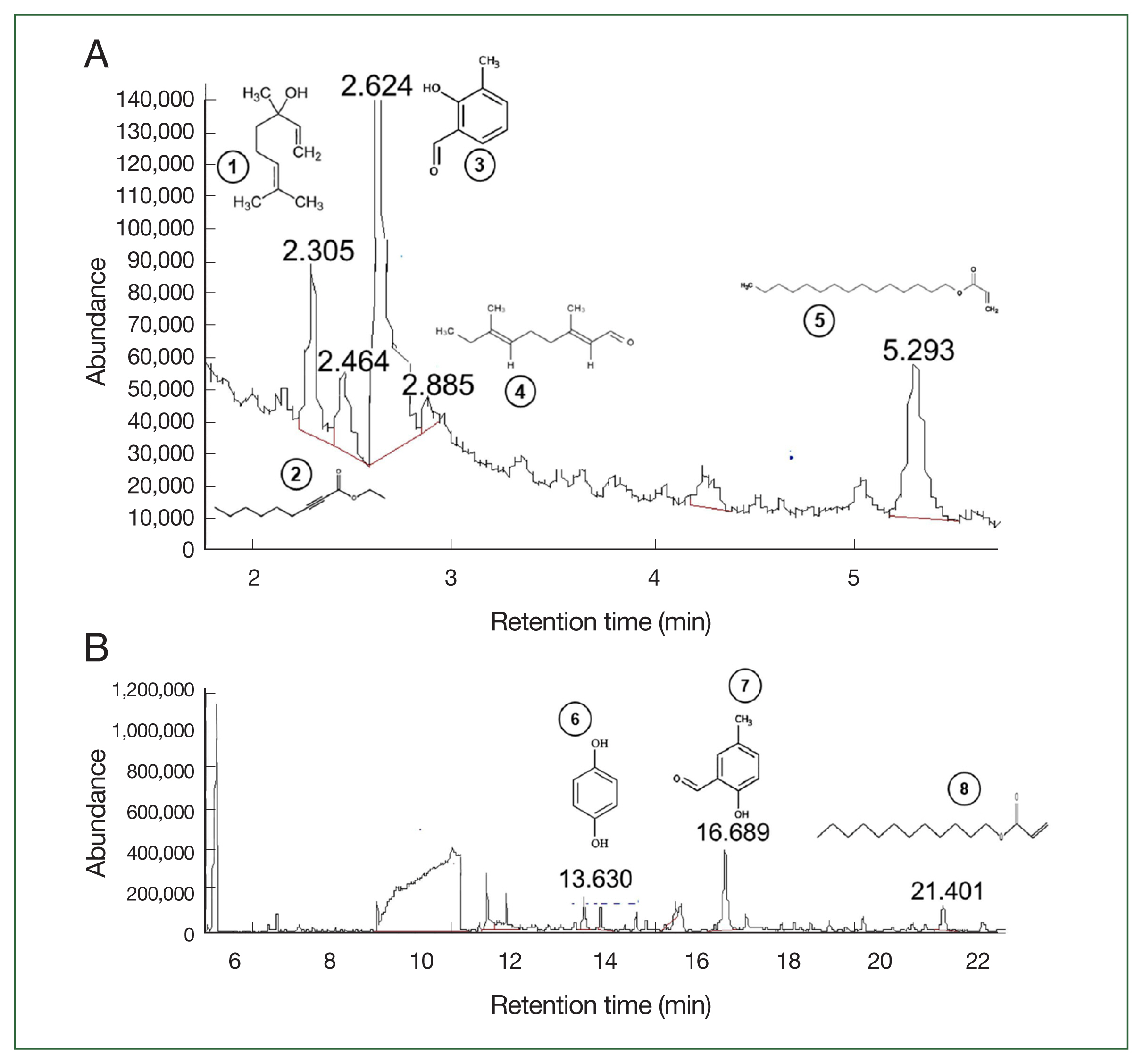
Chromatogram mass spectra of different compounds obtained from the BPFC3MOH1 fraction with their respective retention time and molecular structure. (A) Chromatogram shows the first 5 retention times of the identified compounds. (B) Chromatogram of the last 3 identified compounds. The y-axis shows the relative abundance of each molecule as a function of their mass-charge ratios. Identification of the compound with the retention time (min) for each peak is presented in Table 1.
Anti-Trypanosoma cruzi activity of B. pilosa
The extract and methanolic fraction exhibited higher inhibition percentages against both strains, with inhibitions exceeding 90% at 800 μg/ml concentrations for epimastigotes and trypomastigotes stages. Notably, the crude extract Bp-mOH and fraction BPFC3MOH1 were the most effective, achieving percentages of 100% and 86%, respectively (Fig. 3). The extract and fractions of B. pilosa significantly inhibited T. cruzi, demonstrating a clear dose-response relationship as the percentage of inhibition decreased proportionally with the decrease in extract concentration (Table 2). For both NL and B strains of T. cruzi, complete inhibition was achieved at 800–950 μg/ml concentrations, with inhibitions exceeding 90%. The positive control (100 μg/ml Nifurtimox) exhibited 100% inhibition, while the negative control (culture media with cells only) showed no inhibition. There was no significant difference in the activities of the Bp-mOH extract against NL and B strains of the parasite (P>0.05). Both strains’ LC50 values of the Bp-mOH extract and collective fractions were less than 500 μg/ml (Table 2).
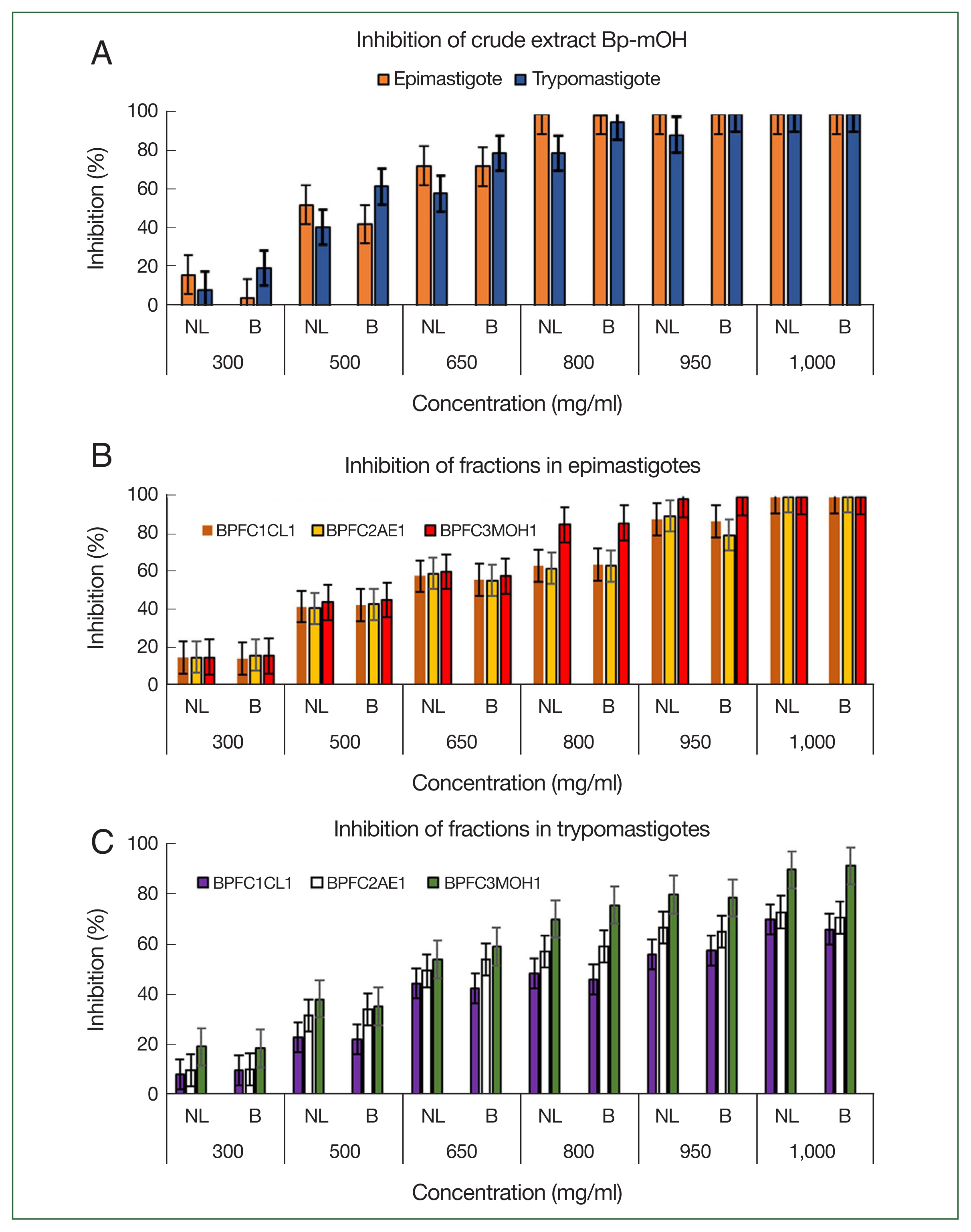
Inhibitory activity (%) of each compound against Trypanosoma cruzi survival. (A) Evaluation of anti-Trypanosoma activity in the presence of crude extract Bp-mOH. (B) Inhibition of fractions in epimastigotes. (C) Inhibition of fractions in trypomastigote stage. The values are shown as the mean of the percent inhibition±SD. Means of NL and B strains are not significantly different based on the Tukey test for multiple comparisons of means (P>0.05). All assays were performed in triplicate.
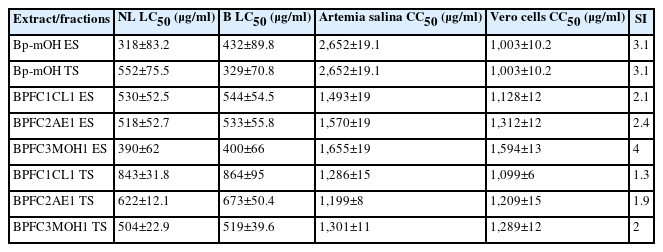
Lethal concentrations (LC50) and cytotoxic concentration (CC50) obtained from epimastigotes and trypomastigotes of A. salina against Vero cells treated with Bp-mOH extract and their fractions
Treatment with the Bp-mOH extract against epimastigote and trypomastigote forms, along with the collective fractions (BPFC1CL1, BPFC2AE, BPFC3MOH1), notably reduced the number of epimastigotes at concentrations greater than 800 μg/ml for the Bp-mOH extract and the number of trypomastigotes at concentrations exceeding 950 μg/ml for the Bp-mOH extract, compared to the positive control (NFX). The percentages of inhibition against epimastigotes and trypomastigotes did not exhibit significant differences, although a higher inhibition was observed against epimastigotes (>95%). However, the effects of collective fractions were less pronounced in terms of the percentage of mortality, resulting in a less significant reduction in the number of parasites, with percentages above 75% (Table 2). The (negative) DMSO control was different from the groups treated with the extracts (P<0.05), indicating that the solvent did not influence the extracts’ anti-trypanosoma activities. Cytotoxic assays were conducted with the Bp-mOH extract and fractions at the same concentrations used for anti-T. cruzi activity, resulting in an LC50 of 318 μg/ml for epimastigotes and 552 μg/ml for trypomastigotes, with similar values observed for both NL and Brener strains. The cytotoxic concentration for 50% of Vero cells (CC50) and the toxic assay with Artemia salina (CC50) indicated a non-cytotoxic effect, with values exceeding 1,000 μg/ml (Table 2).
Hemolysis assay
The hemolytic activity of the Bp-mOH extract, BPFC1CL1, BPFC2AE1, and BPFC3MOH1 fractions was assessed. It was observed that none of the extracts and fractions exhibited significant hemolytic effects. Even at the highest concentration of 3,000 μg/ml, Bp-mOH demonstrated a low hemolysis percentage of 6.4%, while BPFC1CL1, BPFC2AE1, and BPFC3MOH1 caused hemolysis percentages lower than 10%. The negative control did not exhibit hemolysis, while the positive control with distilled water resulted in 100% hemolysis (Table 3). The results of hemolytic activity revealed no hemolysis at concentrations below 500 μg/ml for all the fractions and crude extract. Furthermore, in concentrations exceeding 1,000 μg/ml up to 3,000 μg/ml, no observable hemolysis was detected in the experiments, with percentages remaining below 10%.
Discussion
This study represents the first documentation of the anti-T. cruzi activity of the methanolic extract derived from B. pilosa aerial parts and its fractions. Additionally, it includes the identification of the chemical composition by GC-MS of the fraction exhibiting the highest activity against the flagellated parasite.
The extraction of B. pilosa using methanol (yielding the BPFC3MOH1 fraction) resulted in a notably higher percent yield (26.2%). Interestingly, previous studies had reported lower percentages, often below 8%, which could be attributed to geographical variations or climatic conditions [20–22]. The phytochemical analysis of B. pilosa identified phenolic compounds, alkaloids, carbohydrates, and proteins, consistent with prior research [21]. However, it’s important to note that the plant’s chemical composition can vary based on factors such as the analyzed plant parts, the timing of collection, and the geographic region [22].
The BPFC3MOH1 fraction of B. pilosa underwent analysis via TLC and GC/MS, with the most effective separation of components achieved using methanolic solvents. This analysis revealed chromatographic profiles with signals, retention times, and retention factors similar to those found in other reports that detected these compounds in methanolic extracts from the aerial parts of B. pilosa [21,22]. This study demonstrates that the biological activity against both epimastigotes and trypomastigotes is attributed to the presence of 8 specific compounds. While previous studies had reported antimicrobial [23], antioxidant [24], and antiprotozoal activities [4] for B. pilosa, this research is pioneering in its exploration of anti-T. cruzi activity.
Comparative analyses of the activities of the crude extract and fractions of B. pilosa against NL and B strains of T. cruzi using one-way ANOVA did not reveal significant differences (P>0.05). These results indicate that both strains, despite originating from different lineages and geographic regions, share genomic similarities that make them susceptible to the compounds found in the crude extract and fractions of B. pilosa [25].
The presence of terpenes in B. pilosa leaves, including compounds like 2-furan methanol, 5-ethenyl tetrahydro-α, and α,5-trimethyl-, cis-(linalool), had been reported in various parts of the B. pilosa plant [26]. Linalool, for instance, had been identified among the 66 chemical compounds in the whole plant hexane fraction of Achillea wilhelmsii, exhibiting activity against Leishmania major [27].
The major compounds present in BPFC3MOH1 were 2-hydroxy-3-methylbenzaldehyde (50%), pentadecyl prop-2-enoate (22.1%), and linalool (15.1%). Other, less-abundant compounds included 2-nonynoic acid, ethyl ester (9.3%), 3,7-dimethylnona-2,6-dienal (2.4%, 2-hydroxy-5-methylbenzaldehyde (0.3%), and dodecyl prop-2-enoate (0.1%). It would be interesting to observe each compound’s individual activities to determine if the anti-Trypanosoma activity is due to 2-hydroxy-3-methylbenzalddehyde (the principal sample component), or as the result of some sort of synergic effect that exists among all 8 compounds.
In both strains of T. cruzi, the inhibition percentages at various concentrations for each fraction and the crude extract did not differ significantly. This finding reaffirms the concentrations’ dose-response effect, as previously demonstrated for B. pilosa extracts, which displayed significant antiprotozoal activity against Leishmania amazonensis [4], antimalarial activity [28], and anti-T. brucei rhodesiense in a dose-dependent manner [5]. Various studies have reported these concentration ranges, often falling between 500 μg/ml and over 1,000 μg/ml against various microorganisms. The present results do not categorize these compounds as definitively “good” or “not good,” but they do confirm the low or absent cytotoxic effects compared to other plant extracts [29].
Regarding the toxicity assessments using Artemia salina and the Vero cell line, the CC50 results exceeded 1,000 μg/ml for both the Bp-mOH extract and the 3 evaluated fractions (BPFC1CL1, BPFC2AE1, and BPFC3MOH1). These results indicated that the extract and fractions were non-toxic [29]. Such toxicity studies are paramount, providing comprehensive insights into potential non-harmful effects on different organisms and cells. When results indicate low or no cytotoxicity, they serve as a valuable guide for advancing to more advanced studies, including those involving animal models [30–32].
The Bp-mOH extract exhibited a SI 3.15, while the BPFC3MOH1 fraction displayed an even higher SI (4.03). A higher SI suggests that the fraction will specifically target the parasite while sparing host cells, reducing the likelihood of adverse effects caused by the compound [32,33]. As for the hemolytic activity tests with human erythrocytes using B. pilosa extract and fractions, none displayed visual hemolysis, with hemolysis percentages remaining below 10% across all concentrations used in the experiment. This indicates that these extracts and fractions are nonhemolytic [34]. Currently, challenges in murine models are being assessed with these extracts. Ultimately, this newfound knowledge is hoped to apply to patients with Chagas’ acute or chronic disease, particularly in regions where the incidence has recently surged to at least 50 new cases [35].
In conclusion, our research highlighted the antitrypanosomal properties of B. pilosa, an endemic plant of México, particularly from the Nuevo León region. This plant is also recognized in traditional Mexican herbal medicine for its prophylactic and therapeutic benefits. Methanolic extracts and fractions derived from the aerial parts of B. pilosa, including stems, leaves, and flowers, demonstrated inhibitory effects against both epimastigote and trypomastigote forms of T. cruzi in vitro. Notably, the methanolic fractions were more potent and exhibited no cytotoxicity. Furthermore, the extract and fractions proved nonhemolytic toward human erythrocytes in the experiments. GC-MS analysis identified 8 compounds within the B. pilosa fraction that exhibited the highest trypanocidal activity. These findings not only corroborate the therapeutic potential of Mexican medicinal plants against parasitic infections but also underscore the importance of investigating botanicals as viable sources of novel antiparasitic agents.
Acknowledgments
This research was supported by PAICyT-UANL grant # CN943-19, and 125-CS-2022 (ZJMG and LGS). We are also grateful to CONAHCyT for the scholarship granted to the first author (GECJ) during her Ph.D. degree. We thank to the staff of the Laboratory of Molecular and Experimental Pathology of the FCB for their technical assistance.
Notes
Author contributions
Conceptualization: Molina-Garza ZJ
Data curation: Solís-Soto LY
Formal analysis: Cázares-Jaramillo GE, Molina-Garza ZJ, Luna-Cruz IE, Solís-Soto LY, Galaviz-Silva L
Investigation: Cázares-Jaramillo GE, Molina-Garza ZJ, Solís-Soto LY, Rosales-Encina JL, Galaviz-Silva L
Methodology: Cázares-Jaramillo GE, Solís-Soto LY, Rosales-Encina JL, Galaviz-Silva L
Project administration: Molina-Garza ZJ
Resources: Galaviz-Silva L
Supervision: Molina-Garza ZJ, Luna-Cruz IE
Validation: Luna-Cruz IE, Rosales-Encina JL, Solís-Soto LY
Visualization: Luna-Cruz IE, Rosales-Encina JL, Galaviz-Silva L
Writing – original draft: Cázares-Jaramillo GE
Writing – review & editing: Galaviz-Silva L
The authors declare that there is no conflict of interest regarding the publication of this paper.