Anti-tumor effects of Toxoplasma gondii and antigen-pulsed dendritic cells in mice bearing breast cancer
Article information
Abstract
Cancer immunotherapy is widely used to treat various cancers to augment the weakened host immune response against tumors. Dendritic cells (DCs) are specialized antigen-presenting cells that play dual roles in inducing innate and adaptive immunity. Toxoplasma gondii is a protozoan parasite that exhibits anti-tumor activity against certain types of cancers. However, little is known about the anti-tumor effects of T. gondii or tumor/parasite antigen-pulsed DCs (DC vaccines, DCV) in breast cancer. In this study, C57BL/6 mice were administered E0771 mouse breast cancer cells (Cancer-injected) subcutaneously, T. gondii Me49 cysts orally (TG-injected), or DCs pulsed with breast cancer cell lysate antigen and T. gondii lysate antigens (DCV-injected) intraperitoneally. Tumor size and immunological characteristics were subsequently evaluated. We also evaluated matrix metalloproteinase (MMP)-2 and MMP-9 levels in E0771 mouse breast cancer cells co-cultured with T. gondii or DCs by RT-PCR. The tumor volumes of mice injected with breast cancer cells and antigen-pulsed DCs (Cancer/DCV-injected mice) were similar to those of Cancer-injected mice; however, they were significantly reduced in T. gondii-infected tumor-bearing (TG/Cancer-injected) mice. Moreover, tumor volumes were significantly reduced by adding antigen-pulsed DCs (TG/Cancer/DCV-injected mice) compared to TG/Cancer-injected mice. The levels of IFN-γ, serum IgG2a levels, and CD8+ T cell populations were significantly higher in DCV- and TG-injected mice than in control mice, while no significant differences between Cancer- and Cancer/DCV-injected mice were observed. The levels of IFN-γ, the IgG2a levels, and the percentage of CD8+ T cells were significantly increased in TG/Cancer- and TG/Cancer/DCV-injected mice than in Cancer-injected mice. IFN-γ levels and serum IgG2a levels were further increased in TG/Cancer/DCV-injected mice than in TG/Cancer-injected mice. The MMP-2 and MMP-9 mRNA expressions were significantly decreased in mouse breast cancer cells co-cultured with live T. gondii, T. gondii lysate antigen, or antigen-pulsed DCs (DCV) but not in inactivated DCs. These results indicate that T. gondii induces anti-tumor effects in breast cancer-bearing mice through the induction of strong Th1 immune responses, but not in antigen-pulsed DCs alone. The addition of antigen-pulsed DCs further augments the anti-tumor effects of T. gondii.
Introduction
Breast cancer is one of the most common and challenging malignancies worldwide [1]. Over 2.3 million cases and 685,000 deaths from breast cancer occurred in 2020. By 2040, the burden of breast cancer is predicted to increase to over 3 million new cases and 1 million deaths every year, owing to population growth and aging alone [2]. Although tumor rectomy, radiotherapy, chemotherapy, and hormone therapy are widely used for the treatment of breast cancer, the limitations of these existing treatment regimens have been recognized [3]. Thus, new therapeutic modalities for breast cancer must be developed to eliminate residual circulating cancer cells and micrometastases.
Cancer immunotherapy is a promising approach for combating cancer by restoring the balance of the immune system. Several strategies for cancer immunotherapy have recently been developed [3–5]. Toxoplasma gondii is an intracellular protozoan parasite that chronically infects a wide array of warm-blooded vertebrates following oral ingestion of infectious oocysts or tissue cysts in contaminated soil, water, or food. T. gondii extensively manipulates cellular signaling pathways and host immune responses through secreted effector proteins [6,7]. Thus, T. gondii exerts anti-cancer activity by secreting cancer-associated mucin-type O-glycans, rhoptry proteins, and dense granule antigens that activate the host antigen-presenting system to inhibit tumor growth [7,8]. Several studies have shown that T. gondii induces anti-tumor activity against certain types of cancers; however, limited information is available regarding the effects of T. gondii infection on breast cancer.
Dendritic cells (DCs) are specialized antigen-presenting cells that play dual roles in inducing innate and adaptive immunity. DCs can recognize, process, and present tumor antigens and activate specific T-cell responses that target tumors; thus, they can be used as cellular adjuvants in cancer immunotherapy [3–5]. DC vaccines can be achieved via in vitro culture of autologous DCs along with tumor-associated antigens and are designed to boost the immunogenicity of tumor-associated antigens [3,4]. In addition, vaccination of patients with cancer and autologous or allogeneic tumor cell-based vaccines has proven to be safe and elicit anti-cancer immune responses in clinical trials involving patients with different types of malignancies [5]. Recently, many studies have reported that anti-tumor and antimetastatic actions are associated with DC-based vaccines [3–5,9]. However, there is limited information regarding DC vaccines (antigen-pulsed DCs) in breast cancer immunotherapy.
Despite advancements in conventional therapies, breast cancer is the second leading cause of cancer-related deaths in women in many regions of the world. T. gondii is a powerful agent in cancer immunotherapy and is useful as a stimulant of cellular immune responses. DCs can be used as cellular adjuvants in cancer immunotherapy. However, little is known about the anti-cancer effects of T. gondii or tumor/parasite antigen-pulsed DCs (DC vaccines, DCV) in breast cancer. Therefore, DCs were pulsed with breast cancer cell lysate antigen and T. gondii lysate antigen (TLA) to prepare antigen-pulsed DCs (DCV), and then mice were injected with either E0771 mouse breast cancer cells, T. gondii Me49 cysts, or antigen-pulsed DCs at the indicated time points. The anti-tumor effects were evaluated by tumor size, and immunological mechanisms were assessed by examining cytokine production, serum IgG subclasses, and T-cell phenotypes. We also evaluated matrix metalloproteinase (MMP)-2 and MMP-9 levels in E0771 mouse breast cancer cells co-cultured with T. gondii or DCs by RT-PCR.
Materials and Methods
Ethics statement
All animal experiments were reviewed and approved by the Institutional Animal Care and Use Committee of Chungnam National University College of Medicine (approval No. 202012A-CNU-200).
Cell line
The mouse medullary breast adenocarcinoma cell line, E0771 cell, was obtained from professor Kyu Lim (Chungnam National University College of Medicine, Daejeon, Korea). E0771 cells were originally isolated from spontaneous cancer in C57BL/6 mice [10]. This cell line was cultured in a humidified 5% CO2 incubator at 37°C in Dulbecco’s modified Eagle medium supplemented with 10% heat-inactivated fetal bovine serum (FBS; Gibco/BRL, Grand Island, NY, USA), 100 unit/ml penicillin, 100 μg/ml streptomycin, and 2 mM L-glutamine (Gibco BRL, Grand Island, NY, USA).
Mice
Inbred female C57BL/6 mice were obtained from the Korea Research Institute of Bioscience and Biotechnology (Daejeon, Korea). All mice used were 6–8 weeks old and documented as specific pathogen-free animals. Mice were fed sterilized rodent chow, and water was provided ad libitum.
Preparation of tumor cell antigen
E0771 cells were cultured in 100 mm cell culture dishes. At 90% confluence, flasks were rinsed with phosphate-buffered saline (PBS). Cells were then trypsinized, pelleted, and resuspended in PBS at a concentration of 1×107 cells/ml. The cell suspension was then distributed in 1.5 ml cryotubes. Cells were then frozen by dipping in liquid nitrogen for 30 sec and thawed in 37°C water. This freeze-thaw process was repeated 4 times to increase the immunologic potency of the cells, as observed in previous studies [11]. After cryo treatment, cell viability was determined using a trypan blue exclusion assay. When the lack of cell growth was confirmed, the cryotreated cells were frozen at −80°C until the time of use. Before use, the lysates were thawed and centrifuged at 12,000 rpm for 30 min, and the supernatant was used as the source of the tumor antigen. The concentrations of tumor cell antigens were determined using bovine serum albumin (BSA; Sigma-Aldrich, St. Louis, MO, USA).
Preparation of bone marrow-derived DCs
Bone marrow-derived DCs were generated as described previously [9,12]. Briefly, the tibias and femurs of mice were disinfected with 70% ethanol for 5 min and washed with PBS. Both ends of the bone were cut off with scissors, and the bone marrow cavity was rinsed with sterile PBS using a 1 ml syringe. On day 0, bone marrow cells were seeded at 4×106 cells per 90×15 mm petri dish with 10 ml of complete medium (RPMI 1640 supplemented with 100 μg/ml of antibiotics; Welgene, Gyeongsan, Korea), 10% FBS, and granulocyte-macrophage colony-stimulating factor (20 ng/ml; PeproTech, Rocky Hill, NJ, USA) in combination with IL-4 (1 ng/ml, PeproTech). On day 3, non-adherent granulocytes and T and B cells were gently removed, and a fresh complete medium was added. On day 6, non-adherent and loosely adherent cells were collected by gently pipetting the medium against the plate. The purity of the immature DC population was determined using flow cytometric analysis with anti-mouse CD40, CD80, CD86, and MHC-II monoclonal antibodies (eBioscience, San Diego, CA, USA).
Preparation of antigen-pulsed DCs (DCV) and injection into mice
For the preparation of antigen-pulsed DCs (DCV), immature DCs were incubated with fresh complete media containing tumor cell lysate antigen (100 μg protein/1×106 cell) on day 6. On day 7, TLA (100 μg protein/1×106 cell) was added into the loosely adherent DCs and incubated for 24 h. TLA was prepared from T. gondii RH tachyzoites as described previously [13]. On day 8, the mature DCs were collected and used for immunotherapy.
Four days after the E0771 mouse breast cancer cell injection, 1×106 antigen-pulsed DCs were intraperitoneally injected into pre-infected or normal mice. Two boosters of antigen-pulsed DCs were administered 8 and 12 days after cancer cell injection.
Cancer cell injection into mice and measurement of tumor volumes
E0771 mouse breast cancer cells were harvested via trypsin digestion (1 ml of 0.05% trypsin-ethylenediaminetetraacetic acid) for 5 min, washed with PBS, counted, and diluted with PBS. Breast cancer cells were injected subcutaneously on the right pad of the fourth mammary gland of female C57BL/6 mice with 100 μl of 1.51×106 E0771 cells suspended in PBS using a 22-gauge needle.
Tumor masses were palpable in the subcutaneous tissues 7 days after cancer cell injection. The extent of tumor growth was measured every 3 days using sterile metric calipers. A caliper was used to measure the maximum diameter (tumor length) and minimum diameter (tumor width) of the mouse tumors. Tumor volume was calculated using the following formula: tumor volume (μl)=tumor width (mm)2×tumor length (mm)×0.5 [14].
Experimental design
Mice were randomly divided into 7 experimental groups. Each group was composed of 20 mice; 5 mice were used to evaluate tumor volumes, while 15 mice were used to evaluate immunologic characteristics. The 7 groups were as follows: (1) Control mice: untreated control mice, (2) Cancer-injected mice: mice injected with 1.51×106 E0771 mouse breast cancer cells subcutaneously, (3) DCV-injected mice: mice injected with antigen-pulsed DCs, (4) Cancer/DCV-injected mice: mice injected with breast cancer cells and then injected with antigen-pulsed DCs, (5) TG-injected mice: mice infected with 20 brain cysts of T. gondii Me49 strain orally, (6) TG/Cancer-injected mice: mice infected with T. gondii Me49 strain orally and then injected with breast cancer cells subcutaneously, and (7) TG/Cancer/DCV-injected mice: mice infected with T. gondii Me49 strain orally, injected with breast cancer cells subcutaneously, and then injected with antigen-pulsed DCs. On days 0, 8, and 16 after the cancer cell injection, blood and spleen samples were collected from the mice to evaluate the immunological characteristics of each group (Supplementary Fig. S1). Tumor volumes were measured 7, 10, 13, and 16 days after cancer cell injection. Age- and sex-matched control mice were administered with equivalent doses of normal saline. In all experiments, the general condition of the mice was monitored daily.
Titration of IgG subtypes using ELISA
Each well of a 96-well plate was coated with TLA (10 μg/ml) and incubated overnight at 4°C. After blocking, serum samples were diluted 1:100 in 0.1% BSA/PBS that contained 0.05% Tween-20, and 100 μl of diluted serum sample was added to each well. The plates were incubated for 2 h and HRP-conjugated goat anti-mouse IgG, IgG1, or IgG2a (Southern Biotechnology Associates Inc., Birmingham, AL, USA) was added. Following the final washing step, freshly prepared O-phenylenediamine dihydrochloride was added, and the reaction was stopped by adding 4 N H2SO4. Optical density was measured at 492 nm using an automated ELISA reader (Tecan A-5082, Salzburg, Austria).
Splenocyte preparation and flow cytometry
Following removal from the mice, the spleens were homogenized, and erythrocytes were lysed in RBC lysis buffer (Sigma-Aldrich). Splenocytes (1×106 cells) were incubated with 50 μl of anti-mouse CD4+ BV421 and CD8+ Apc-cy7 monoclonal antibodies or an isotype-specific control (PharMingen, San Diego, CA, USA. 1:100 dilution in 0.1% BSA/PBS) for 15 min at 4°C. The cells were washed 3 times in 0.1% BSA/PBS using centrifugation and analyzed using flow cytometry (ACEA Biosciences, CA, USA). All data were analyzed using the FlowJo Software (Treestar, Ashland, OR, USA).
In vitro culture of mouse splenocytes and cytokine assay
Murine splenocytes were cultured in RPMI 1640 medium supplemented with 10% FBS, 2 mM L-glutamine, 25 mM HEPES, and antibiotics-antimycotics at 37°C and 5% CO2. Culture supernatants were harvested 3 and 5 days after incubation, and then IL-10 and IFN-γ levels of each group were measured using commercial ELISA kits following the manufacturer’s instructions (R&D Systems, Minneapolis, MN, USA). Cytokine concentrations were determined by reference to standard curves constructed with known amounts of mouse recombinant IL-10 or IFN-γ. The sensitivity limits for the assays were 1.97 pg/mL for IL-10 and 2 pg/ml for IFN-γ.
RT-PCR for the detection of MMPs
E0771 mouse breast cancer cells (5×104/chamber) were plated in the lower upper parts of Transwell (Corning Coaster, Cambridge, MA, USA). The upper chambers were loaded with inactivated DCs (2×105/chamber), antigen-pulsed DCs (DCV, 2×105/chamber), live T. gondii tachyzoites (1×106/chamber), or TLA (50 μg/ml). The cells were incubated for 24 h in a 5% CO2 incubator. The cells in the lower chamber were collected to determine MMP-2 and MMP-9 mRNA expression levels by RT-PCR.
mRNA expressions of MMPs were assessed according to the method described by Gui and Fan [15]. Total mRNA was isolated using the RNAgent kit (Promega, Madison, WI, USA). The cDNA was prepared using a Reverse Transcriptase Premix kit (Cat. EBT-1515). PCR was performed using 2× Taq PCR Pre-MIX kit (STD02-P096) and 0.4 μm each of the 3′-primer and 5′-primer in a total volume of 50 μl. The cycling conditions were as follows: initial denaturation at 94°C for 30 sec, followed by 30 cycles of denaturation at 94°C for 30 sec, annealing at 55°C for 30 sec, and extension at 72°C 30 sec. The primer sequences were as followings: MMP-2 forward: 5′-AGATCTGCAAACAGGACATTGTATT-3′ and reverse: 5′-TTCTTCTTCACCTCATTGTATCTCC-3′; MMP-9 forward: 5′-CTGGGCTTAGATCATTCCTCAGT-3′ and reverse: 5′-AGTACTTCCCATCCTTGAACAAATA-3′; β-actin forward: 5′-AGCACAATGAAGATCAAGAT-3′ and reverse: 5′-TGTAACGCAACTAAGTCATA-3′. Amplification was linear within the range of 20–30 cycles. PCR products were resolved on a 2% agarose gel and stained with SYBR Green I (Molecular Probes, Eugene, OR, USA).
Statistical analysis
All results are expressed as the mean±SD for each group. To evaluate differences in tumor sizes, cytokine levels, antibody titers, and phenotype profiles, Kruskal-Wallis tests were used to check for statistical differences among the groups. Differences between groups were considered significant at P<0.05.
Results
T. gondii infection/antigen-pulsed DCs inhibited tumor growth in breast cancer-bearing mice
Tumor masses were palpable in the subcutaneous tissues of Cancer-injected mice 5 days after cancer cell injection. The skin overlying the tumor began to ulcerate 10 days after cancer cell injection in Cancer- and Cancer/DCV-injected mice, whereas ulceration was observed from day 13 in TG/Cancer- and TG/Cancer/DCV-injected mice (Fig. 1A). The tumor volumes of Cancer/DCV-injected mice were similar to those of Cancer-injected mice. In the Cancer- and Cancer/DCV-injected mice, tumor masses were increased abruptly in size from day 7 after cancer cell injection, and the tumor volumes at day 16 were 1,727±165 and 1,453±136 μl, respectively. The tumor volumes were significantly inhibited in the TG/Cancer- and TG/Cancer/DCV-injected mice (834±102 μl and 587±93 μl, respectively) at day 16 after cancer cell injection as compared to the Cancer- or Cancer/DCV-injected mice (P<0.05). Moreover, tumor volumes were significantly reduced by adding antigen-pulsed DCs (TG/Cancer/DCV-injected mice) compared to TG/Cancer-injected mice (P<0.05, Fig. 1B).
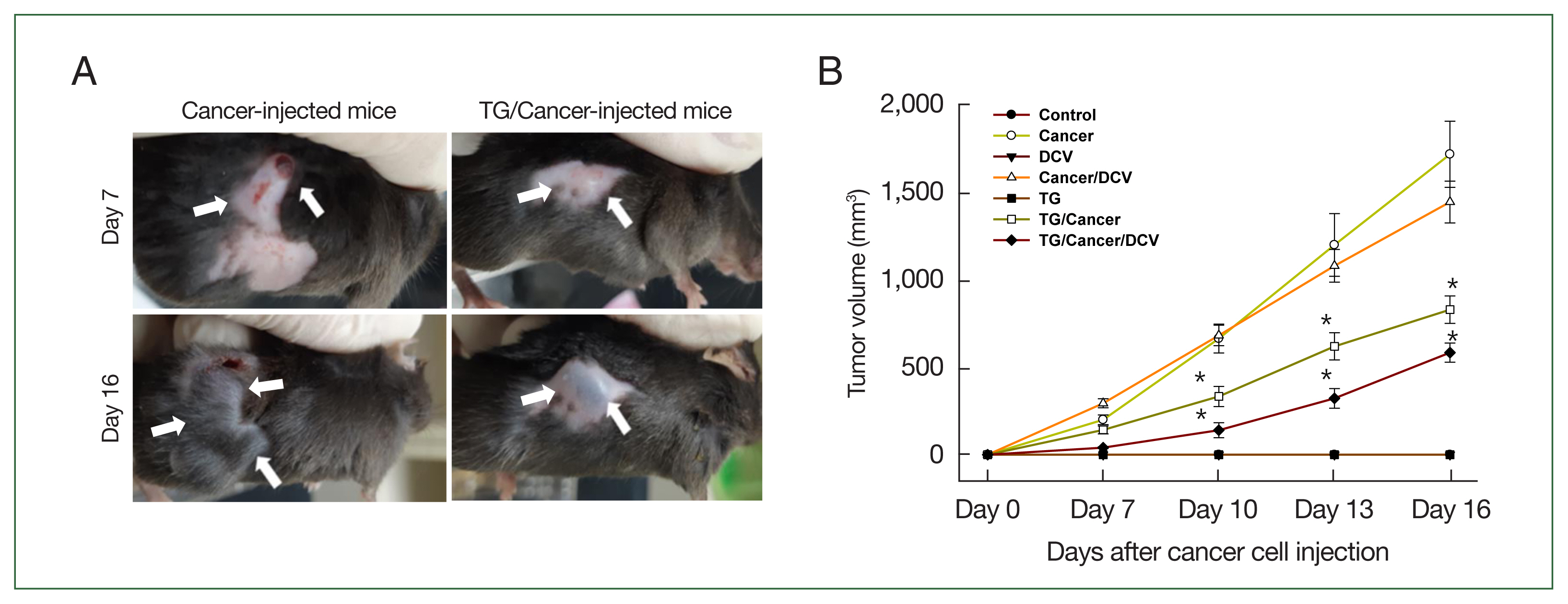
Sizes of tumors in C57BL/6 mice injected with breast cancer cells (Cancer), Toxoplasma gondii (TG), or antigen-pulsed dendritic cells (DC vaccine, DCV). Tumor growth was measured every 3 days from 1 week after cancer cell injection using metric calipers. Tumor volume (μl)=tumor width (mm)2×tumor length (mm)×0.5. (A) Tumor mass of Cancer- and TG-injected mice at days 7 and 16 after cancer cell injection, (B) tumor volumes of each group according to different injection formulas. Data are expressed as mean±SD of 5 mice. Control, untreated control mice; Cancer, injection with E0771 mouse breast cancer cells subcutaneously; DCV, injection with antigen-pulsed dendritic cells intraperitoneally; Cancer/DCV, mice injected with cancer cells and antigen-pulsed DCs; TG, infection with T. gondii Me49 cysts orally; TG/Cancer, mice infected with T. gondii and then injected with cancer cells; and TG/Cancer/DCV, mice infected with T. gondii, and then injected with cancer cells and antigen-pulse DCs. *P<0.05 compared with Cancer-or Cancer/DCV-injected group.
IFN-γ levels were increased in T. gondii-infected breast cancer-bearing mice
The levels of IFN-γ production in the Cancer- and Cancer/DCV-injected mice were similar to those in the control mice. However, IFN-γ levels were significantly increased in the DCV-, TG-, TG/Cancer-, or TG/Cancer/DCV-injected mice as compared to control mice (P<0.05). The levels of IFN-γ were markedly increased at 16 days after cancer cell injection in TG/Cancer/DCV-injected mice (Fig. 2A).
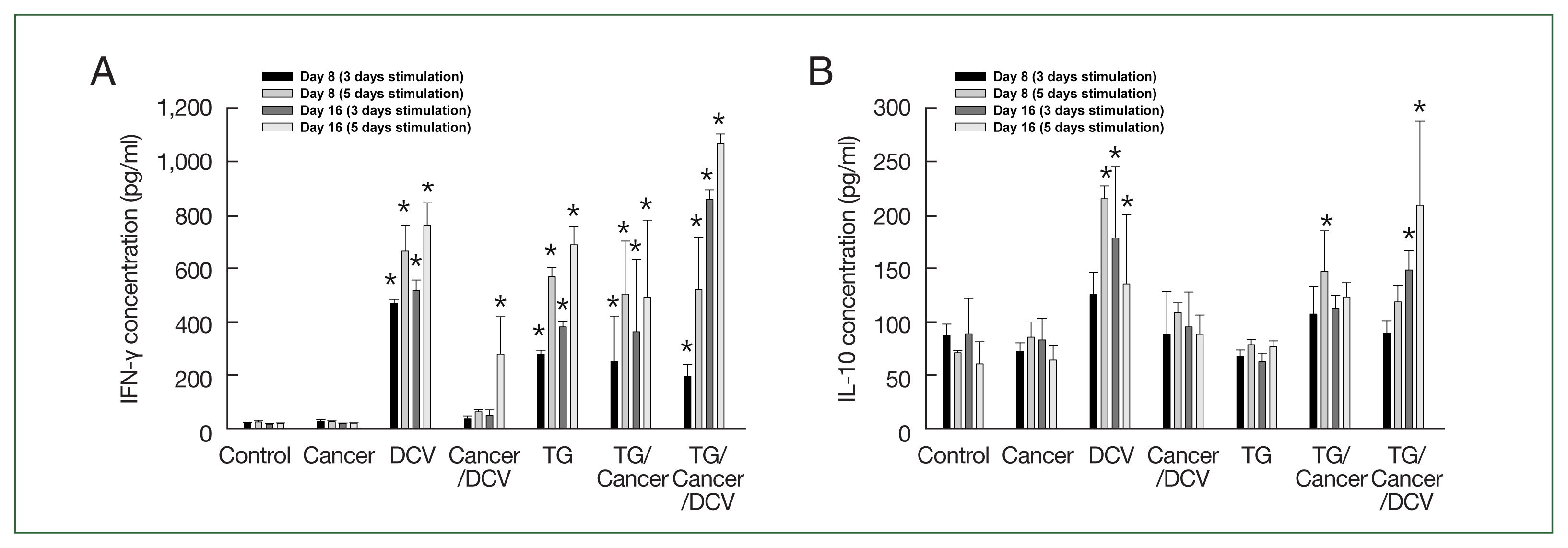
Cytokine production in the splenocytes from C57BL/6 mice injected with breast cancer cells, Toxoplasma gondii, or antigen-pulsed dendritic cells. Splenocytes were harvested at the time points indicated and then the concentration of (A) IFN-γ and (B) IL-10 levels in the culture supernatants of splenocytes were evaluated using ELISA after incubating for 3 days and 5 days. Data are expressed as mean±SD of 5 mice. Control, untreated control mice; Cancer, injection with E0771 mouse breast cancer cells subcutaneously; DCV, injection with antigen-pulsed dendritic cells intraperitoneally; Cancer/DCV, mice injected with cancer cells and antigen-pulsed dendritic cells; TG, infection with T. gondii Me49 cysts orally; TG/Cancer, mice infected with T. gondii and then injected with cancer cells; and TG/Cancer/DCV, mice infected with T. gondii, and then injected with cancer cells and antigen-pulsed dendritic cells. *P<0.05 compared with Control or Cancer-injected group.
The levels of IL-10 production in the Cancer-, Cancer/DCV-, TG-, and TG/Cancer-injected mice were not significantly different from those in the control mice. However, the levels of IL-10 in DCV- and TG/Cancer/DCV-injected mice were significantly higher than those in control mice on day 16 after cancer cell injection (P<0.05, Fig. 2B).
IgG2a levels, but not IgG1 levels were increased in T. gondii-infected breast cancer-bearing mice
The IgG, IgG1, and IgG2a titers of Cancer- and Cancer/DCV-injected mice were similar to those of the control mice. The IgG titers of TG-, TG/Cancer-, and TG/Cancer/DCV-injected mice were significantly higher than those of the control mice from day 8 after cancer cell injection (P<0.05, Fig. 3A). The IgG1 titers of TG-, TG/Cancer-, and DCV-injected mice were not significantly different from those of control mice (Fig. 3B). However, the IgG2a titers of the DCV-, TG-, TG/Cancer-, and TG/Cancer/DCV-injected mice significantly increased on days 8 and 16 after cancer cell injection. In particular, the IgG2a titers of the TG/Cancer/DCV-injected mice were significantly higher than those of the DCV-, TG-, and TG/Cancer-injected mice (Fig. 3C).
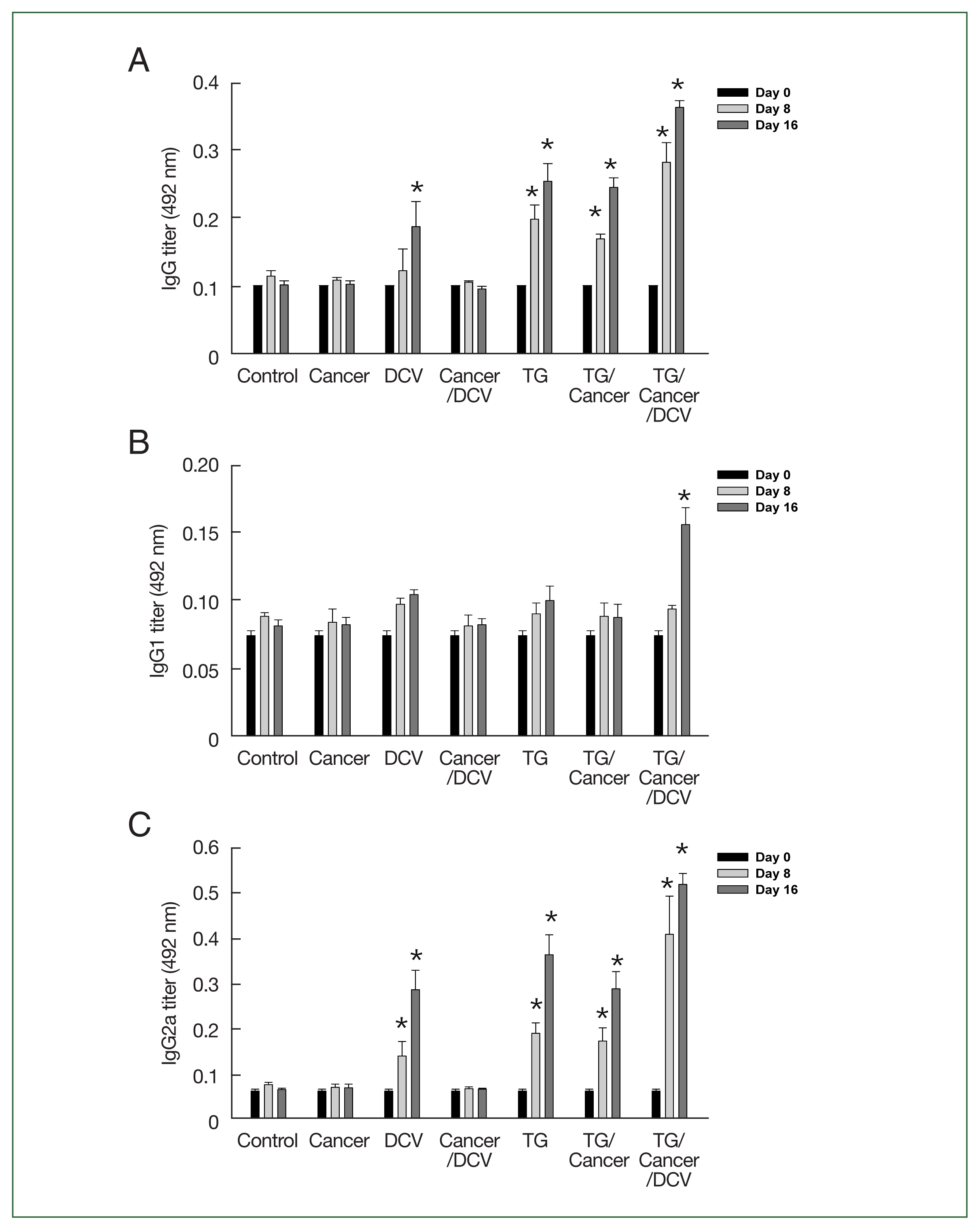
Specific antibody production in C57BL/6 mice injected with breast cancer cells, Toxoplasma gondii, or antigen-pulsed DCs. Serum samples were obtained from mice at the indicated time points, and then (A) IgG, (B) IgG1, and (C) IgG2a titers of each group were measured at 0, 8, and 16 days after cancer cell injection using ELISA. Data are expressed as the mean±SD of 5 mice. Control, untreated control mice; Cancer, injection with E0771 mouse breast cancer cells subcutaneously; DCV, injection with antigen-pulsed dendritic cells intraperitoneally; Cancer/DCV, mice injected with cancer cells and antigen-pulsed dendritic cells; TG, infection with T. gondii Me49 cysts orally; TG/Cancer, mice infected with T. gondii and then injected with cancer cells; and TG/Cancer/DCV, mice infected with T. gondii, and then injected with cancer cells and antigen-pulsed dendritic cells. *P<0.05 compared with control or Cancer-injected group.
Percentages of CD8+ T cells were augmented in T. gondii-infected breast cancer-bearing mice
To evaluate the changes in T-cell phenotypes following the injection of cancer cells, T. gondii infection, or antigen-pulsed DCs, we analyzed the percentage of splenic CD4+ and CD8+ T cells using flow cytometry (Fig. 4A). As shown in Fig. 4B, the percentages of CD8+ T cells among splenic T cells in Cancer- and Cancer/DCV-injected mice were similar to those in control mice. However, the percentage of CD8+ T cells was significantly increased in DCV-, TG-, TG/Cancer-, and TG/Cancer/DCV-injected mice on days 8 and 16 after injection compared to control mice (P<0.05).
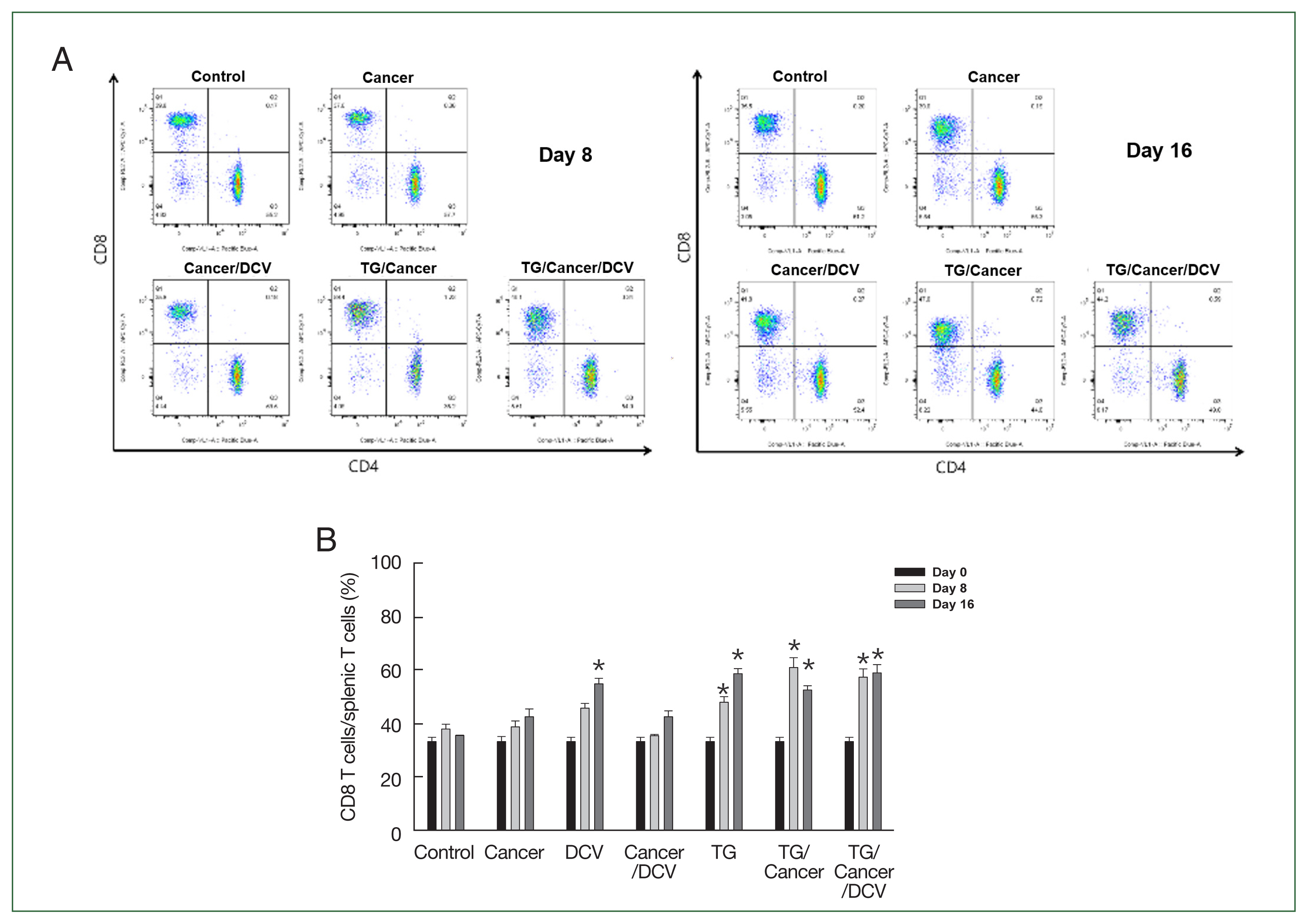
Percentages of CD8+ T cells from C57BL/6 mice injected with breast cancer cells, Toxoplasma gondii, or antigen-pulsed dendritic cells. Splenocytes were stained with 50 μl of anti-mouse CD4+ BV421 and CD8+ Apc-cy7 monoclonal antibodies. (A) The percentages of CD4+ and CD8+ T cells were then evaluated using flow cytometry at days 0, 8, and 16 after cancer cell injection. (B) The percentages of splenic CD8+ T cells subpopulations. Data are expressed as the mean±SD of 5 mice. Control, untreated control mice; Cancer, injection with E0771 mouse breast cancer cells subcutaneously; DCV, injection with antigen-pulsed dendritic cells intraperitoneally; Cancer/DCV, mice injected with cancer cells and antigen-pulsed dendritic cells; TG, infection with T. gondii Me49 cysts orally; TG/Cancer, mice infected with T. gondii and then injected with cancer cells; and TG/Cancer/DCV, mice infected with T. gondii, and then injected with cancer cells and antigen-pulsed dendritic cells. *P<0.05 compared with control or Cancer-injected group.
MMP-2 and MMP-9 expression levels were inhibited in E0771 mouse breast cancer cells co-cultured with live T. gondii and antigen-pulsed DCs
Cell motility, a critical process for invasion and metastasis of primary tumors, is primarily driven by MMPs, especially MMP-2 and MMP-9 [16,17]. The levels of MMP-2 and MMP-9 mRNA expression were significantly inhibited in the breast cancer cells co-cultured with antigen-pulsed DCs (DCV), live T. gondii (TG), or TLA for 24 h (Fig. 5). However, there were n significant changes in MMP-2 and MMP-9 mRNA expression levels in breast cancer cells co-cultured with inactivated DCs compared to untreated control cells.
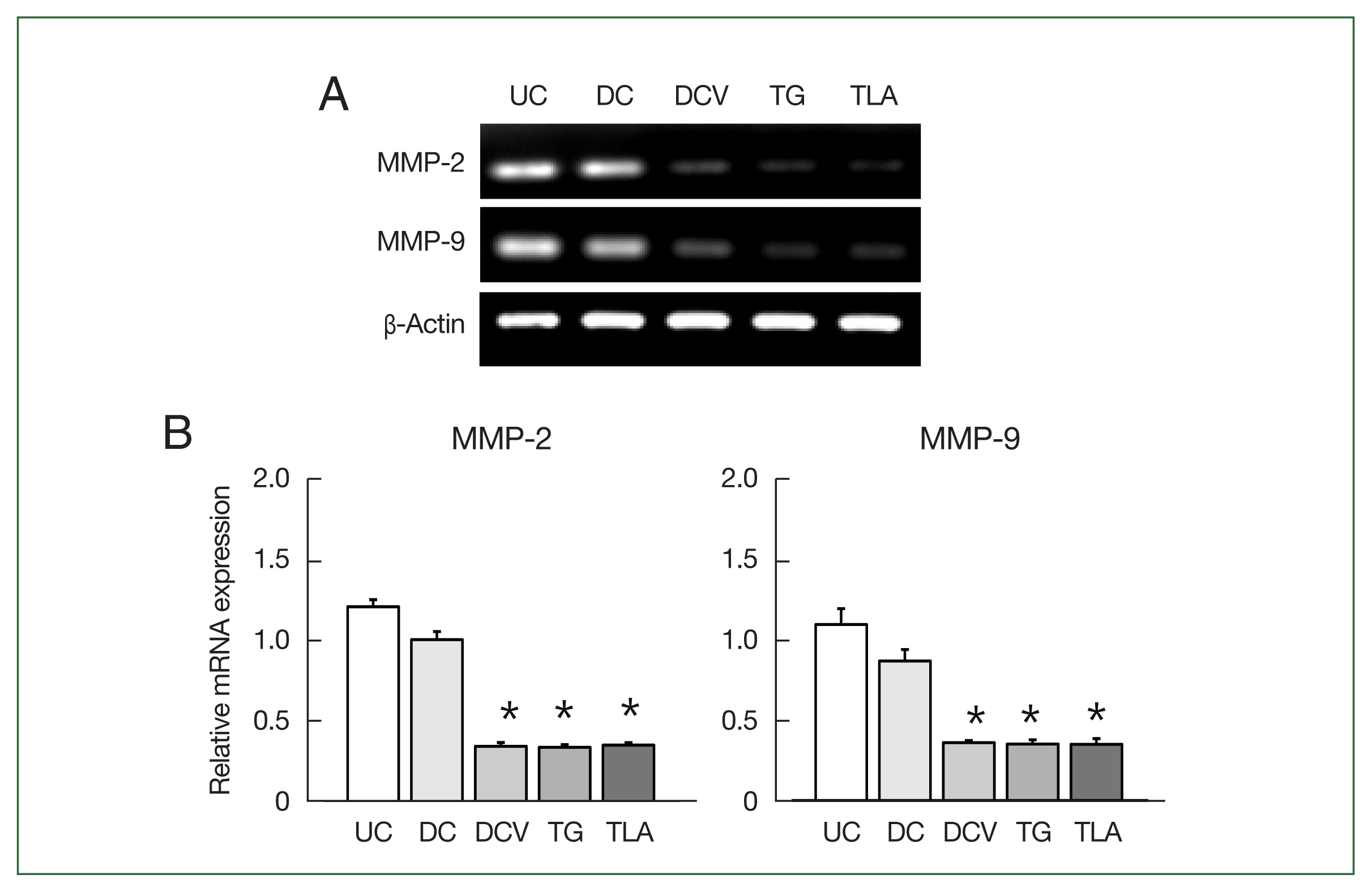
Matrix metalloproteinase (MMP)-2 and MMP-9 levels in breast cancer cells stimulated with dendritic cells or Toxoplasma gondii. E0771 mouse breast cancer cells were co-cultured with inactivated dendritic cells (DC), antigen-pulsed dendritic cells (DCV), live T. gondii (TG) or Toxoplasma lysate antigen (TLA) for 24 h, and then the levels of MMP-2 and MMP-9 mRNA expressions were measured by RT-PCR. PCR products were resolved on a 2% agarose gel (A), and the MMP-2 and MMP-9 mRNA levels were quantified (B). Data are expressed as the mean±SD of 5 samples. *P<0.05 compared with untreated control (UC) group.
Discussion
Cancer immunotherapy strengthens host immune response against tumors using specific and nonspecific immune stimulants. Various pathogens have been long used in cancer immunotherapy [18]. T. gondii is a powerful agent for cancer immunotherapy and is useful as a stimulant of cellular immune responses [6–9]. DCs are a promising target for activating the immune system in immunotherapeutic strategies against cancer [3–5]. Therefore, we evaluated the anti-cancer effects of T. gondii and antigen-pulsed DCs (DCV) in breast cancer model mice. We found that tumor volumes were significantly decreased in TG/Cancer- and TG/Cancer/DCV-injected mice than in Cancer- and Cancer/DCV-injected mice. These data indicate that T. gondii induced strong anti-tumor effects in the mouse model of breast cancer, similar to that reported by Xu et al. [19]. They reported that the avirulent uracil auxotroph T. gondii stimulated anti-infection and anti-tumor immunity in mice, inhibiting tumor growth and metastasis in 4T1 murine breast cancer [19]. A recent study revealed that T. gondii DNA is present in the malignant breast tissues of humans; thus, human anti-T. gondii IgG was beneficial for breast cancer survival [20].
The efficiency of the anti-tumor response may be significantly influenced by the targeted migration of effector T cells to the tumor site as guided by the DC vaccination route. Administration methods for DC vaccines include intravenous injection, direct injection into the tumor site, and intraperitoneal injection, and so on. In our study, the DC vaccine was administered intraperitoneally, which is a widely recognized method [21–24]. Interestingly, intraperitoneal immunization results in superior antitumor immune response and tumor suppression compared with subcutaneous and intratumoral administration of DC vaccine in a KPC mouse model of pancreatic ductal adenocarcinoma [21]. Based on the specific interplay between T. gondii and DCs, the different DC subsets likely display distinct responses to T. gondii infection in terms of cytokine responses, antigen presentation, and parasite clearance [25,26]. In addition, exosomes derived from DCs infected with T. gondii could be used for developing novel cancer therapeutic strategies by reducing the proportion of myeloid-derived suppressor cells in a mouse model of colorectal cancer [27]. In this study, we used the antigen-pulsed DCs (DCV) prepared using inactivated DCs stimulated with TLA and tumor antigen, to evaluate the anti-cancer effects in breast cancer model mice. In the current study, tumor volumes were reduced in Cancer/DCV-injected mice than in Cancer-injected mice, however the difference was not significant. However, tumor volumes were significantly decreased in TG/Cancer/DCV-injected mice than in Cancer-, Cancer/DCV-, and TG/Cancer-injected mice. These findings indicate that antigen-pulsed DCs alone do not have effective anti-cancer effects but can be used as a cellular adjuvant for cancer immunotherapy to augment the anti-tumor effects of T. gondii.
We also investigated the immunological mechanisms underlying the anti-tumor activity of T. gondii and antigen-pulsed DCs in breast cancer. Cytokines are critical cell-mediated immunity regulators that work through enhancing and limiting immune responses [28]. CD4+ T cells are divided into 2 classes based on the range of cytokines produced. Th1 cells produce IL-2 and IFN-γ and control the production of IgG2a, whereas Th2 cells produce IL-4, IL-5, and IL-10 and control the production of IgG1 and IgE. Th1 cells promote cell-mediated immunity, whereas Th2 cells induce B cell differentiation and inhibit cell-mediated immunity [28]. In the current study, the levels of IFN-γ and IgG2a titers were significantly higher in DCV- or TG-injected mice than in control mice. Furthermore, their levels were significantly higher in TG/Cancer-injected mice than in Cancer- or Cancer/DCV-injected mice and their levels of TG/Cancer/DCV-injected mice increased significantly than those of TG/Cancer-injected mice. These results indicated that T. gondii induces strong Th1 immune responses in breast cancer, and the addition of antigen-pulsed DCs further augmented these effects. Our results are consistent with those of previous reports suggesting that the induction of Th1 immune responses, characterized by high IFN-γ production and IgG2a titers, can inhibit the proliferation of malignant cells [6–8,14,19]. The secretion of specific proteins by T. gondii in conjunction with host cell invasion activates anti-tumor immunity through host responses involving CD8α+ DCs, the IL-12/IFN-γ Th1 axis, as well as CD4+ and CD8+ T cells [7,8,14,19]. In the present study, the percentage of CD8+ T cells was significantly higher in TG-injected mice than in control mice from day 8 after injection, and the percentages of CD8+ T cells were significantly higher in TG/Cancer- and TG/Cancer/DCV-injected mice than in Cancer- or Cancer/DCV-injected mice. These data suggest that T. gondii provides an appropriate epitope for MHC-1 presentation and induces high percentages of CD8+ T-cells.
This study evaluated the anti-cancer effects of T. gondii or DCs in in vitro systems. Proteolytic enzymes play important roles in cancer development and metastasis. Particularly, MMP-2 and MMP-9 play important roles in breast cancer metastasis by degrading the extracellular matrix and basement membrane to facilitate the invasion and migration of cancer cells [16,17]. We found that live T. gondii, TLA, and antigen-pulsed DCs (DCV) significantly inhibited the expressions of MMP-2 and MMP-9 in breast cancer cells, whereas inactivated DCs did not suppress MMP-2 and MMP-9 expressions. These data indicated that T. gondii and antigen-pulsed DCs are associated with suppressing the tumor progression and invasion in breast cancer cells. From the above results, we confirmed that T. gondii exhibits anti-tumor effects both in vivo and in vitro and that its effects are augmented by the addition of antigen-pulsed DCs.
In this study, we established the anticancer effects of T. gondii; however, several limitations remain. Further research is needed to determine the specific molecules of T. gondii responsible for its anticancer effect. In addition, when T. gondii and antigen-pulsed DCs (DC vaccine) are administered together, investigation into its mechanism of action and potential side effects are required more in detail. Furthermore, the applicability of the DC vaccine prepared in study in actual clinical practice is also essential. In summary, our results suggest that T. gondii induces an anti-tumor effect in breast cancer-bearing mice through the induction of strong Th1-type immune responses, characterized by increased IFN-γ production, IgG2a titers, and CD8+ T-cell percentages. The anti-tumor effects of T. gondii were further augmented by the addition of antigen-pulsed DCs. These results highlight the potential of T. gondii-derived factors as promising candidates for novel novel immunotherapies against breast cancer.
Notes
Author contributions
Conceptualization: Lee YH
Data curation: Kim BK, Choi HG, Lee YH
Formal analysis: Kim BK, Choi HG, Lee YH
Funding acquisition: Lee YH
Investigation: Kim BK, Choi HG, Lee JH, Cha GH, Lee YH
Methodology: Kim BK, Choi HG, Lee JH, Choi IW
Software: Kim BK, Choi HG, Lee JH, Choi IW
Supervision: Yuk JM, Cha GH
Validation: Yuk JM, Cha GH
Writing – original draft: Lee YH
Conflict of interest
The authors declare no conflict of interest related to this study.
Acknowledgments
This work was supported by the National Research Foundation of Korea (NRF) grant funded by the Korea government (MIST) (2022R1A2B5B01001641).
Supplementary Information
Supplementary material is available with this article at https//doi.org/10.3347/PHD.24082.