Immune Responses of NIH Mice Infected with Avirulent and Virulent Strains of Plasmodium chabaudi adami Single and Mixed Infections
Article information
Abstract
An understanding of the nature of the immune response to asexual erythrocytic stages of malaria parasites will facilitate vaccine development by identifying which responses the vaccine should preferentially induce. The present study examined and compared the immune responses of NIH mice in either single or mixed infections with avirulent (DK) or virulent (DS) strains of Plasmodium chabaudi adami using the ELISA test for detecting and measurement of cytokines and antibody production. In both single and mixed infections, the study showed that both cell- and antibody-mediated responses were activated. In all experiments, an early relatively high level of IFN-γ and IgG2a during the acute phase of the infection, and later elevation of IL-4 and IgG1, suggested that there was a sequential Th1/Th2 response. However, in the avirulent DK strain infection a stronger Th1 response was observed compared to the virulent DS strain-infection or in mixed infections. In the virulent DS infection, there was a stronger Th2 response compared to that in the DK and mixed infections. The faster proliferation rate of the virulent DS strain compared to the DK strain was also evident.
INTRODUCTION
Malaria is the most important parasitic human disease which kills between 1.5 and 2.7 million people in endemic areas [1]. Asexual blood stages of malaria parasites are responsible for all the morbidity and mortality of the disease [2]. A better understanding of the immune responses against these stages will be an important step towards development of effective vaccines to reduce clinical disease and prevent death. For this purpose, findings from experimental studies on both single and mixed infections are useful because in humans mixtures of different species and different genotypes of the same species of malaria parasites are common in malaria-endemic regions [3].
Acquired immunity to the asexual blood stages of malaria in mice involves both cell-mediated and antibody-dependent mechanisms through activation of 2 subsets of CD4+ T cells, i.e., Th1 and Th2 cells [4]. In a self resolving P. chabaudi AS infection, there was a sequential Th1/Th2 response in which the Th1 subset responsible to control the acute phase of the patent parasitemia while Th2 response rises when infection becomes chronic [5,6] and could play a role in the elimination of the infection [7]. In a virulent infection of P. yoelii, a failure to induce adequate activation of both Th1 and Th2 subsets resulted in a fatal outcome [8]. In susceptible A/J mice, spleen cells produce a lower level of IFN-γ and early higher levels of IL-4 and IL-10 compared to infection in resistant B6 mice in P. chabaudi AS infections [9,10].
It is known that IFN-γ is central to the induction of IgG2a production which indicates a Th1 response while IL-4 indicates production of IgG1 and Th2 activation [11]. IFN-γ plays an important role in resistance to asexual blood stages of malaria via activated macrophages which kill parasites through phagocytosis and by release of reactive oxygen molecules which have been shown to destroy intraerythrocytic P. yoelii in vitro [12]. Moreover, IFN-γ gene knockout (GKO) C57BL/6 mice infected with P. chabaudi AS had higher morbidity and mortality compared to controls [13-15].
In mice infected with P. chabaudi AS, IgG2a is a predominant antibody during the acute primary parasitemia followed by a rise in IgG1 in the chronic phase as a result of CD4+ Th1 to Th2 switching [16,17]. Smith and Taylor-Robinson [6] compared the humoral response in inbred NIH mice infected with pairs of parasites from virulent and avirulent strains; lethal P. chabaudi 7/F1 with non-lethal P. chabaudi AS, and lethal P. yoelii 17XL with non-lethal P. yoelii 17XNL strains. Their results showed that in non-lethal infections there was an early significant up-regulation of IgG2a followed by later IgG1 production while in lethal infections the IgG2a levels were slower and reduced which would result in a rapid fatal outcome before IgG1 levels significantly synthesized [6].
Inducing both Th1 and Th2 responses through immunization is an important aim for malaria control because both humoral and cellular responses are required for resolution of infection [18,19]. Both IFN-γ and IL-4 responses are produced when BALB/c mice were immunized with a genomic P. chabaudi adami DS expression library constructs and challenged with P. chabaudi adami DS [20] as a stringer model for evaluation of vaccine efficacy [21,22].
Mixed malaria infection studies in both humans and rodents provide information on the kinetics of the course of infection, the impact on clinical outcome [23], and the immune responses induced compared with that in single strain species infections. The course and pathological effects of a single infection may be altered by the presence of another species [24,25]. Epidemiological studies suggested that a Plasmodium vivax infection might ameliorate a subsequent infection with Plasmodium falciparum [26]. Cross-species immunity in murine malaria parasites [27,28] and the specificity of clearance mechanisms of the acute primary parasitemia have also been shown [29,30]. Studies of the dynamics of mixed infection using a DNA hybridization assay showed that when CBA/Ca mice were simultaneously inoculated with a mixture of cloned lines of P. chabaudi chabaudi and P. yoelii no deaths were observed compared to the control groups infected with a single line of each parasite in which low but consistent mortality was observed [25]. These results show that the outcome of mixed infections is influenced by the composition of the infecting parasite populations. In mixed infections when a species or genotype-specific response cleared the majority population [31], the minority populations could later expand [32].
The present study determined and compared the immune responses, cross-immunity, and inter-reaction between 2 malaria parasite strains, the DK and the DS strains of P. chabaudi adami which differ in virulence, in a resistant rodent host, the female NIH mouse in both single and mixed infections. The influence of virulence and the effect of a low infective dose of the DS strain on the course of single or mixed infections were also studied.
MATERIALS AND METHODS
Mice
Female NIH mice (Harlan Laboratories, Bicester, UK) were kept in the Joint Animal Facility, Glasgow University, and used when they were at about 6-8 weeks of age. If it was required, mice were kept in a reverse light/dark cycle, with light from 19 : 00 to 07 : 00. They were fed with standard diet (Beekay Universal Ltd, Alborough, UK) before infection and on diet CRM (S. & S. Scotland Ltd, UK) after infection.
Parasites
Avirulent, P. chabaudi adami DK, and virulent P. chabaudi adami DS strains (courtesy of the late Professor David Walliker, University of Edinburgh, UK) were used. The capsule of the cryopreserved infected blood was taken from liquid N2 and thawed in a 37℃ water bath (Gallencamp, Daresbury, UK) or in the hand. To the thawed blood, an equal volume of 0.3 ml 17% sorbitol, was mixed and then inoculated intraperitoneally (i.p.) into a mouse. Parasites were then maintained if required by sub-passage through susceptible mice. Briefly, infected mice were sacrificed in a CO2 chamber and bled by cardiac puncture and into a syringe containing sodium heparin at 10 i.u. heparin per ml of blood. The parasitemia of the donor mouse was determined by examination of a Giemsa (Gurr BDH Ltd, Dorset, UK) stained thin blood smear. The infected blood was subsequently diluted to give the required infective doses of the parasitized red blood cells (pRBCs). Here, infective doses were 1 × 104 pRBCs for P. chabaudi adami DS, 1 × 105 pRBCs for P. chabaudi adami DK, and a mixture of 2 × 103 pRBCs of DS plus 8 × 103 pRBCs of DK (1 × 104 total) for mixed infection in 0.25 ml PBS which were inoculated intravenously (i.v.). Non-infected red blood cells were added to the diluent of PBS to avoid spontaneous lysis of pRBCs at a very low concentration of RBCs.
Chloroquine treatment
Sub-curative dose of chloroquine (chloroquine disulphate salt, Sigma, St. Louis, Missouri, USA), 24 mg/kg was given i.p. to the mice infected with virulent DS parasites.
Determination of parasitemia
Parasitemia was determined by examination of Giemsa stained thin blood smears collected daily from sample mice and viewed under oil immersion (Gurr BDH).
Presentation of parasitemia
On each day, the parasitemia was determined for each group as both the mean percentage (at least RBCs of 50 fields at each microscopy were counted) and the geometric mean (mean log10 pRBCs per 105 RBCs).
Collection of serum
Mice were bled by cardiac puncture and blood was allowed to clot at room temperature (RT) and was then put into the cold room for at least 1 hr to give better clot contraction. Contaminating RBCs was separated by centrifugation for 5 min at 6,000 g. Normally the sample was then aliquoted into 20 µl volumes in small Eppendorf tubes (Greiner, Stonehouse, UK), labelled, and stored at -20℃ until required. For small serum samples, blood was collected (up to 100 µl) from the tail.
Spleen cell culture for proliferation and cytokine assays
Spleen cells were cultured as described by Daneshvar et al. [33]. The aseptically removed spleen was teased gently in a Petri dish (Greiner) in RPMI-1640 and splenocytes were harvested with a sterile Pasteur pipette. All separated spleen cell suspensions were centrifuged, 10 min at 1,200 g at 4℃, resuspended in 0.3 ml of Boyle's solution to lyse erythrocytes. After 3 washes with RPMI or PBS, the pellets were resuspended in 10 ml of complete RPMI-1640. The number of viable splenocytes was calculated by diluting 10 µl of washed spleen cells in 190 µl of 0.2% trypan blue (Sigma) in PBS using a Neubauer Haematocytometer under phase contrast microscope (Diavert Leitz, Berlin, Germany). The cell concentrations were adjusted to 5 × 106 cells/ml per each well with the final volume of 200 µl and transferred into triplicate wells of sterile 96-well flat-bottomed tissue culture plates (IWAKI, Tokyo, Japan). The whole prepared spleen cell suspensions from infected or naïve mice were separately cultured with pRBCs as antigens, RBCs, medium, or concanavalin A (Con A, Sigma) as controls. For the pRBCs and the RBCs the total RBCs was the same. Con A was added at 0.5 µg/well. The plates were then incubated for 72 hr in 5% CO2 in air in a 37℃ incubator (Flow laboratory, Thame, UK). The supernatants from each well were then collected and stored at -20℃ until required.
For the splenocytes proliferation assay, a separate plate was prepared as above, but it was first incubated for 60 hr and 0.5 µCi (37KBq) [3H] thymidine (Amersham, Little Chalfront, UK) was then added to each well, and the plate was incubated for a further 12-18 hr. The cells were harvested on a printed filtermat (WALLC, Finland) by a harvester machine (Tomtec, Hamden, Connecticut, USA). Uptake of [3H] thymidine was measured using liquid scintillation (Opstiscint Hisafe, Wallac, England) by a β-counter and presented as counts per minute (c.p.m). Whole spleen cell suspensions from infected or naïve mice were separately cultured in medium of RPMI-1640 (Gibco Life Technologies, Paisley, UK) supplemented with 10% fetal calf serum (FCS) as complete medium, (Labtech International, Ringmer, UK) with pRBCs, RBCs, medium, or Con A (Sigma). Con A was added at 5 µg/ml (final concentration was 0.5 µg/well) as a positive control and complete RPMI-1640 medium was used as negative control.
Preparation of pRBCs and RBCs lysates
Mice were kept in reverse light so that the asexual erythrocytic cycle was reversed and could be collected in the late morning when the parasites were mostly at the late ring/trophozoite stage. P. chabaudi adami DS and DK strain antigens were prepared as crude antigens from whole blood cells enriched for mature trophozoite/schizont pRBCs, using the method described by McDonald & Sherman [34]. Briefly, infected heparinized (10 i.u./ml) blood was cultured in vitro from ring to trophozoite/schizont stage using the candle jar method of Trager and Jensen [35]. The parasites were then harvested, washed in 5% FCS RPMI-1640 medium (200 g for 10 min), resuspended to their original volume and run through Whatman CF11 columns to remove leukocytes. The filtrate was washed with PBS and was restored in PBS to blood volume and then freeze-thawed 5 times by snap freezing the blood cells in liquid N2 and then immediately defrosting in a waterbath at 37℃ to fracture the RBCs and release of the parasites' components. The freeze-thawed preparation was centrifuged at 1,500 g for 10 min, and the supernatant, which is called lysate, was stored in 50 µl aliquots at -20℃ until required. Lysates from non-infected red blood cells as a control and from mixed infection were similarly prepared. Protein concentration of lysates was measured using Coomassie Protein Assay reagent (Pierce, Rockford, Illinois, USA)
Cytokine analysis by ELISA
Purified anti-mouse cytokines, i.e., IFN-γ, IL-4 (PharMingen, San Diego, California, USA) monoclonal antibodies, were diluted in 0.1 M NaHCO3 pH 8.2 as a coating buffer at 2 µg/ml. For each sample, triplicate wells were used. The antibody solution was added in 50 µl to each well in a 96-well plate, covered, and incubated overnight at 4℃ in the cold room. The plates were subsequently washed 3 times with 0.05% Tween in PBS pH 7.2, and then blocked with 10% FCS PBS at 200 µl per each well, covered, and incubated for 1 hr at 37℃. After blocking, the plate was washed 2 times with 0.05% Tween/PBS. Serial dilutions of recombinant cytokine standards (for IFN-γ stock was at 1 mg/ml, and for IL-4 stock was at 0.5 mg/ml, PharMingen) and the culture supernatants were added to the wells at 50 µl/well, covered, and incubated at 37℃ for 3 hr. After incubation, the plate was washed 4 times with 0.05% Tween/PBS, and 50 µl/well of biotinylated rat anti-mouse cytokine (IFN-γ and IL-4, at 2 µg/ml, PharMingen) in 10% FCS PBS were added, covered, and incubated at 37℃ for 1 hr. After washing 6 times, diluted streptavadin-peroxidase, 100 µl/well in 10% FCS PBS, were added. The plate was covered, and incubated for 1 hr at 37℃. The plate was then washed 8 times with 0.05% Tween/PBS, and 100 µl/well of 3,3',5,5'-tetramethylbenzidine (TMB Microwell™, KPL, Gaithersburg, Maryland, USA) substrate was added and the colour allowed to develop. The plate was read at 630 nm with reference filter at 405 nm using Biolinx Dynatech software (Dynatech Laboratoies, Roseville, Canada).
Determination of specific antimalarial IgG (whole, IgG1, and IgG2a)
The same procedure was used for IgGs detection. However, lysates of pRBCs and RBCs (control) were diluted to 20 µg/ml and used as antigen. Total anti-mouse IgG (whole molecule) was diluted at 1 : 100,000. For IgG1 or IgG2a, biotinylated and the secondary antibodies were diluted to 2 µg/ml in 10% FCS.
Statistical analysis
The results presented here are expressed as standard error of mean (± SEM) for each set of values in each experiment. However, SEM cannot be shown when it is too small. When comparison between 2 variables at the same time point is considered, Student's t-tests were performed. When data were compared between more than 2 groups, two-way or one-way ANOVA, and their post tests, were performed. A significant result was considered when the P value was < 0.05.
RESULTS
Parasitemia
In P. chabaudi adami DK-infected mice, the parasitemia peaked at 23.9% on day 10 post-infection (PI) and then quickly decreased to sub-patent levels on day 15 PI. A recrudescent parasitemia was seen between days 38 and 43 PI. In the DS infected mice treated with subcurative dose of chloroquine on day 8 PI, the peak parasitemia was observed on day 8 PI with a maximum of 46.1% and declined to sub-patent levels on day 15 PI. A recrudescence was detected between days 18 and 24 PI.
In untreated mice infected with the DS, the parasitemia peaked at 68.7% on day 10 PI which was significantly higher than that in the treated group (t-test; P < 0.005). In surviving mice, a recrudescent parasitemia was observed between days 21 and 25 PI (Fig. 1). No deaths were observed in avirulent DK infection and in the virulent DS infection when mice were choloroquine treated. However, in untreated DS-infected mice, 60% of mice died.
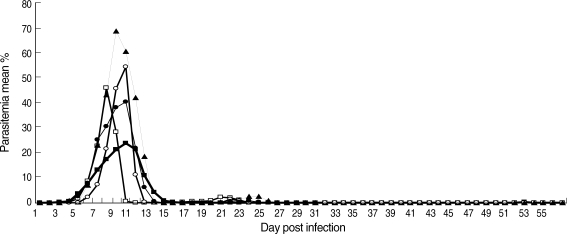
The course of malaria infections in NIH mice. (■) Infective dose: 1 × 105 pRBCs of P. chabaudi adami DK; (□) Infective dose: 1 × 104 pRBCs of P. chabaudi DS and mice treated with chloroquine on day 8 PI; (▲) Infective dose: 1 × 104 pRBCs of P. chabaudi DS in mice left untreated; (●) Mixed infection: a mixture of 8 × 103 pRBCs of P. chabaudi adami DK, and 2 × 103 pRBCs of P. chabaudi DS; (○) Infective dose: 2 × 103 pRBCs of P. chabaudi DS for low dose infection. Sample size was 21 mice for the single infections, 18 mice for the mixed infection and 6 for the low dose infection. Each data point is a mean % parasitemia. For each time point, ± SEM has been calculated. However, SEM cannot be shown when it is too small. The peak parasitemia was 23.9% on day 10 PI for DK-infected mice. The DS-infected mice were treated with a subcurative dose of chloroquine at 24 mg/kg on day 8 PI.
In the low infective dose DS-infection group, infected with 2 × 103 pRBCs, the peak parasitemia was 54.5% on day 10 PI which was significantly (t-test; P < 0.005) lower than that in mice infected with a higher infective dose. In addition, the lower infective dose extended the prepatent period by 1 day (Fig. 1). The surviving rate was 33% compared with the 40% in higher infective dose infections.
The parasitemia in the mixed infection peaked at 40.3% on day 10 PI (Fig. 1). A recrudescence was observed between days 20 and day 26 PI. The survival rate was 100%. There was a significantly lower (t-test; P < 0.0001) parasitemia in the mixed infections compared to the single infections of P. chabaudi adami DS, when mice were left untreated. Despite a relatively small proportion of the virulent DS parasites in the mixed infective dose, the parasitemia peaked significantly (t-test; P < 0.0001) higher than that in the single-infections with avirulent DK strain. Although the number of pRBCs of P. chabaudi adami DS inoculated in the mixed infections and in the single infections with a low infective dose was the same (2 × 103), the peak parasitemia in mice infected with a single low infective dose was significantly higher (t-test; P < 0.0045) than that in the mixed infections (data not shown).
Splenocyte proliferative responses
In both the single and mixed infections, the evaluation of proliferative responses showed that the spleen cells from naive mice which were exposed to Con A had the highest (Tukey's tëst; P < 0.0001 for the DK, P < 0.0002 for the DS strain) levels compared to all other groups for all time points during the experiments. Over all for all time points, the second highest responses were observed in splenocytes from infected mice exposed to Con A and the third highest levels of responses were seen for splenocytes from infected mice, which were re-stimulated with pRBCs (data not shown).
Stimulation index (SI)
In general, the lower SIs were observed after resolution of the peak parasitemia and they rose again after the recrudescent parasitemia compare to other time points. However, at the peak parasitemia, the proliferative responses were low compared to other time points. Splenocytes from mice, infected with the virulent DS and chloroquine treated, showed the lowest SIs compared to the other groups.
IFN-γ production
Supernatants of splenocyte cultures were used to measure the cytokines, IFN-γ and IL-4. As shown in Fig. 2, in the avirulent DK strain infection, there was an early production of IFN-γ with the highest level on the first sampling day, day 4 PI, and it remained at high levels until the peak parasitemia occurred. On day 11 PI, a day after the peak parasitemia, IFN-γ declined sharply but gradually increased thereafter, particularly after the recrudescence. The levels of IFN-γ in splenocyte cultures of infected mice were significantly higher (Tukey's test; P < 0.0001) compared to levels in naive control mice on the first sampling day.
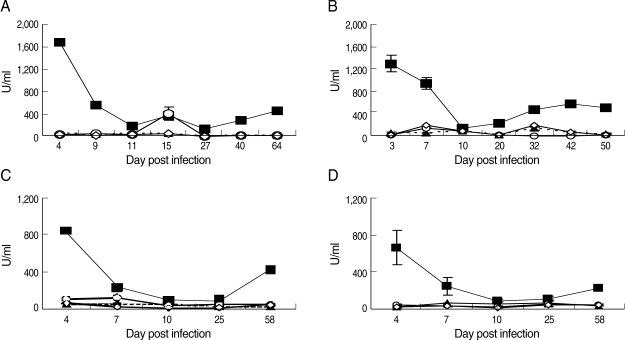
IFN-γ production in NIH mice infected with different strains of P. chabaudi adami DK and DS. (A) Mice infected with 1 × 105 pRBCs of DK strain; (B) Mice infected with 1 × 104 pRBCs of DS and treated with chloroquine; (C) Mice infected with 1 × 104 pRBCs of DS and left untreated; (D) Mice infected with a mixture of 8 × 103 pRBCs of P. chabaudi adami DK, and 2 × 103 pRBCs of P. chabaudi DS. Supernatant of splenocytes cultures for each mouse was assayed separately by ELISA. Each data point is the mean ± SEM for an experiment performed in triplicate. However, SEM is not shown when it is too small. (■) Splenocytes from infected mice exposed to parasitized RBCs (pRBCs); (▲) Splenocytes from naive mice exposed to pRBCs; (○) Splenocytes from infected mice exposed to nRBCs; (◇) Splenocytes from naive mice exposed to nRBCs.
In virulent DS infections, the same profile was seen. The highest levels of IFN-γ were seen on the first sampling day, day 3 and 4 PI in treated and untreated mice, respectively, and the lowest levels were coincident with the peak parasitemia (Fig. 2). The profile of early production of IFN-γ in the mixed infection was the same as in the single-infections. However, the IFN-γ level on the first sampling day was significantly lower (Tukey's test; P < 0.001) than that in mice infected with the DK strain and in the mice infected with virulent DS infection (Tukey's test; P < 0.0001) (Fig. 2).
IL-4 production
In both single and mixed infections, IL-4 levels in supernatants of splenocyte cultures increased later during the observation period (Fig. 3). In the early period of observation, in both virulent and avirulent single infections, IL-4 did not increase above background control levels. The highest levels of IL-4 were detected on the last sampling days in both the DK and DS infections which were significantly higher (Tukey's test; P < 0.001 for the DK infection, P < 0.0001 for the untreated and treated DS infections, and P < 0.0001 for mixed infection) compared to the IL-4 levels of controls for all other time points (Fig. 3). The level of IL-4 in the mixed infection was significantly lower than that in the single infections of DS strain in either untreated (Tukey's test; P < 0.001) or treated mice (Tukey's test; P < 0.001).
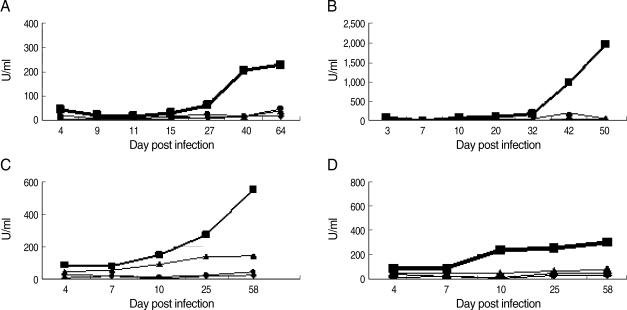
IL-4 production in NIH mice infected different strains of P. chabaudi adami DK and DS. (A) Mice infected with 1 × 105 pRBCs of DK strain; (B) Mice infected with 1 × 104 pRBCs of DS and treated with chloroquine; (C) Mice infected with 1 × 104 pRBCs of DS and left untreated; (D) Mice infected with a mixture of 8 × 103 pRBCs of P. chabaudi adami DK, and 2 × 103 pRBCs of P. chabaudi DS. Supernatant of splenocyte cultures for each mouse was assayed separately by ELISA. Each data point is the mean ± SEM for an experiment performed in triplicate. However, SEM is not shown when it is too small. (■) Splenocytes from infected mice exposed to parasitized RBCs (pRBCs); (▲) Splenocytes from naive mice exposed to pRBCs; (○) Splenocytes from infected mice exposed to nRBCs; (◇) Splenocytes from naive mice exposed to nRBCs.
Production of specific antimalarial IgG (total), IgG1, and IgG2a
The same profile for IgG (whole molecule; total), IgG1, and IgG2a antibody production was generally seen in the DK, DS, and mixed infections. IgG antibody (whole molecule) increased at or immediately after the peak parasitemia, declined thereafter, and then increased after the recrudescence, usually with the highest levels on the last sampling days (data not shown).
In both single and mixed infections, specific IgG1 did not increase significantly during the acute phase, but increased after the recrudescence and subsequently remained at high levels during the observation period (Fig. 4). The levels of IgG1 on the last sampling days were significantly (Tukey's test; P < 0.05 for all groups) higher compared with the control groups. In mice infected with a lower DS infective dose the production of IgG1 was not as high as that seen in other experimental groups of DS-infected mice such as those infected with a higher infective dose.
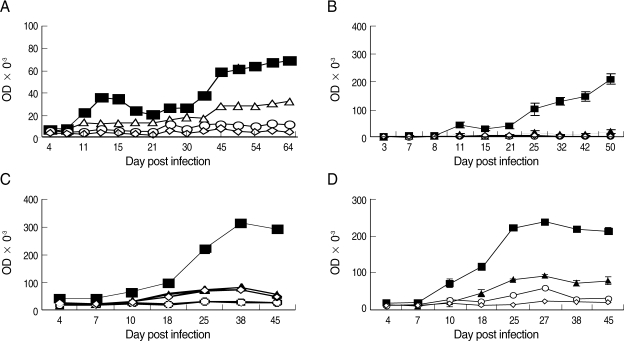
Specific anti-parasite IgG1 in NIH mice infected with different strains of P. chabaudi adami DK and DS. (A) Mice infected with 1 × 105 pRBCs of DK strain; (B) Mice infected with 1 × 104 pRBCs of DS and treated with chloroquine; (C) Mice infected with 1 × 104 pRBCs of DS and left untreated; (D) Mice infected with a mixture of 8 × 103 pRBCs of P. chabaudi adami DK, and 2 × 103 pRBCs of P. chabaudi adami DS. Sera were collected at different time points for ELISA tests. Each data point is the mean ± SEM for an experiment performed in triplicate. However, SEM is not shown when it is too small. (■) Sera from infected mice with DK or DS strains and reacted with the lysate of pRBCs of P. chabaudi adami DK or DS; (▲) Sera from DK- or DS-infected mice reacted with the lysate of RBCs of naÏve mice; (○) Sera from naÏve mice reacted with the lysate of pRBCs of DK or DS strains; (◇) Sera from naÏve mice reacted to the lysate of RBCs from naÏve control mice.
In general, specific-IgG2a sharply increased at or immediately after the peak parasitemia, declined thereafter, and rose again during the later stages of the observation period in all groups. There were higher levels of IgG2a production in mice surviving in the untreated DS-infected group compared to the DK-infected mice or treated DS-infected mice for all time points from day 7 PI onward. There were significantly (Tukey's test; P < 0.0001) higher levels of IgG2a in the mixed infection compared to the DK strain infection and in treated mice infected with the DS strain (Tukey's test; P < 0.0004) (Fig. 5).
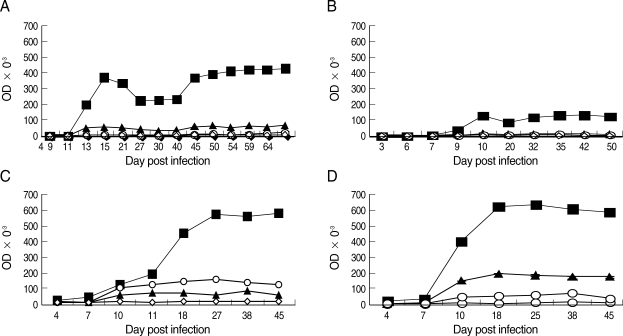
Specific anti-parasite IgG2a in NIH mice infected with P. chabaudi adami DK and DS strains. (A) Mice infected with 1 × 105 pRBCs of DK strain; (B) Mice infected with 1 × 104 pRBCs of DS and treated with chloroquine; (C) Mice infected with 1 × 104 pRBCs of DS and left untreated; (D) Mice infected with a mixture of 8 × 103 pRBCs of P. chabaudi adami DK, and 2 × 103 pRBCs of P. chabaud adami DS. Sera were collected at different time points for ELISA tests. Each data point is the mean ± SEM for an experiment performed in triplicate. However, SEM is not shown when it is too small. (■) Sera from infected mice with DK or DS strains and reacted with the lysate of pRBCs of P. chabaudi adami DK or DS. (▲) Sera from DK-or DS-infected mice reacted with the lysate of RBCs of naÏve mice. (○) Sera from naÏve mice reacted with the lysate of pRBCs of DK or DS strains. (◇) Sera from naÏve mice reacted to the lysate of RBCs from naÏve control mice.
DISCUSSION
In the present study, investigation and comparison of the profile of immune responses in NIH mice single or mixed-infected with avirulent DK and virulent DS strains was the main aim. Therefore, the course of infection, production and kinetics of selected cytokines, and parasite-specific antibodies were examined.
In the DK and DS single and mixed infections, including mice infected with a lower infective dose of DS pRBCs, peak parasitemia was close to the same day post-infection, and this is despite the fact that, in the virulent DS-infected mice, the infective dose was 10-fold lower than that in the avirulent DK infection. In mice infected with the DS strain, a range of mortality between 96.7% and 36% has been reported [22]. Our results showed 60% mortality in untreated mice infected with 1 × 104 pRBCs, and 34% when the mice were infected with a lower infective dose of 2 × 103 pRBCs, and no deaths were recorded in the mixed infections. A reduced infective dose of 2 × 103 pRBCs of DS, resulted in 1-day delay in the appearance of a patent parasitemia and a 3-day delay in the time taken to death compared to infection with the higher infective dose. This suggests that, in the virulent DS infection, the parasite density increases more rapidly due to a higher replication rate compared with the avirulent DK strain infection.
In the mixed infection, the peak parasitemia was significantly higher than that in the DK strain infection but significantly lower than that in the untreated mice infected with the DS strain. This suggests that the presence of the avirulent DK strain may partially moderate the virulence of the DS infection. Similarly, Snounou et al. [25] showed that in the presence of P. chabaudi chabaudi CB the parasitemia of P. chabaudi chabaudi AS and P. chabaudi adami DS was reduced. Cox and Voller [27] also indicated cross-immunity reactions between rodent species of Plasmodium.
A relatively large infective dose results in developing more quickly symptoms in mice [36] and in humans [37]. Thus, Timms and colleagues [38] reported that C57BL/6J mice infected with the virulent P. chabaudi chabaudi BC died about a day earlier for every 10-fold increase in the infective dose. The size of the infective dose also affects Th1 and Th2 sequential activation. Taylor-Robinson and Phillips [39] showed that increasing the infective dose of P. chabaudi chabaudi AS in susceptible A/J mice results in elevated Th2 responses that lead to a fulminant parasitemia whereas increasing the infective dose in resistant NIH mice enhances IFN-γ and reduces IL-4 production promoting a Th1 response. Our observations also support the idea that dose affects disease severity because the survival rates were higher in low dose DS-infected mice compared with high dose DS-infection. The amelioration in the severity of disease in low dose infected mice could be due to giving mice more time to mobilize the immune response before the threshold for clinical disease is reached [38,40]. We, however, observed no alteration in the profile of the immune response in resistant NIH mice in either single or mixed infections of DK or DS strains of P. chabaudi adami.
We showed that recovery from the primary peak parasitemia during a single P. chabaudi adami DK and DS or DK and DS mixed infections in NIH mice is associated with a Th1 response, with high levels of IFN-γ [41] and high levels of specific IgG2a antibody at or immediately after the peak parasitemia. The predominant Th1 cell-mediated response was followed by a Th2 response with high levels of IL-4 and specific IgG1 antibody, particularly on the last sampling days which was coincident with the chronic phase of the infection. This suggests that sequential Th1/Th2 response are induced. Our findings are in agreement with previous studies in which both Th1 and Th2 subsets [42,43] are crucial for the resolution of the acute primary parasitemia in the P. chabaudi adami model [44] and in a self-resolving infection of P. chabaudi AS [4,45].
In respect of IL-4 production, in the avirulent DK infection, the IL-4 levels were significantly lower than those in the virulent DS infections, an indicative of stronger Th2 response in DS-infected mice compared to DK-infected mice. In the mixed infection, also higher levels of IL-4 were recorded on the first sampling day compared to the single infections with DK or DS strains in untreated mice. Therefore, it may be proposed that there was a stronger Th1 response in the single infection while a Th2 response was relatively stronger in the mixed infection. Our observation of increased IL-4 and specific IgG1 antibody levels later in our experiments was also in agreement with those who have suggested that there is a switching from a Th1 to a Th2 response in some murine malaria parasite infections [43,45]. This switching could happen when IL-4 initiates to rise as an anti-inflammatory cytokines, such as IFN-γ [46].
We also observed that the co-presence of the avirulent DK strain with the DS strain in the mixed infection moderates the virulence of the virulent strain. In this regard, although the non-immunological effects or exact interactions between DK and DS strains regarding development of the immune response are not clear, there are some possible explanations. A non-immunological possibility could be competition between the 2 strains for essential nutrients for growth, such as para-aminobenzoic acid.
Some immunological possibilities could be as follows: First, the presence of DK strain can activate, as described before, Th1 response dependent mechanisms such as specific IgG2a production or non-specific macrophage activation, production of nitric oxide or oxygen radicals. These mechanisms as they control the DK infection may also have a bystander effect on DS parasites and apparently to reduce the virulence of the DS strain and thereby, the severity of disease might be ameliorated. Secondly, cross-reactions could be another possibility as indicated by Cox and Voller [27] between rodent malaria parasites. In the present study, it may be supposed that when the immune system develops specific responses to the antigens of DK strain these responses non-specifically have some effective cross-reactions against the shared antigens in the DS strain parasites. Therefore, in the mixed infection, a lower peak parasitemia and no deaths were observed compared with the DS single infection. It is also possible that priming with DK antigens could promote specific responses to the DS strain.
Our study showed much more rapid parasite load of the DS strain and its greater virulence compared with the DK strain infection when a mixed infective dose was administered at a fixed ratio. Therefore, further studies in which different ratios should be used may provide better understanding of interactions between the 2 strains.
We observed that an early rise in IFN-γ with a sharp decline at the peak parasitemia was followed by another rise later during the infection. Therefore, as some previous studies [2,47], we assumed that stimulation of non-specific immunity may be a source of the early rise in IFN-γ measured on day 4. It, therefore, appears that the immune response in P. chabaudi adami DS and DK starts to balance inflammatory and anti-inflammatory cytokines around the time of peak parasitemia because the rise in IL-4 begins after the peak. Nevertheless, understanding which mechanisms and effector cells are involved in the decrease of IFN-γ in splenocytes taken at the time of the peak parasitemia merits more investigations.
The Th1-Th2 cytokine balance influences the regulation of IgG2a and IgG1 isotypes [48]. In lethal infections, there is a correlation between fatal outcome and slower, reduced specific Ig-G2a antibody [6]. However, our results showed that the levels of IgG2a relatively increased again later during the course of the DS infection in surviving mice besides elevation of specific IgG1 antibody.
We showed that IL-4 and igG1 increased later in the course of infections in both avirulent and virulent infections as a marker for switching from Th1 to Th2 response [5,45]. The same observation was indicated by Taylor-Robinson and Phillips [16] in the infection with P. chabaudi chabaudi AS in NIH. Our results are in agreement with von der Weid et al. [49] and van der Heyde et al. [50] who suggested that the presence of IL-4 is not essential for the primary control of parasitemia. IL-4 deficient mice recovered from P. chabaudi and P. yoelii infections, whereas, in IFN-γ receptor deficient mice, the primary parasitemia failed to decline to a sub-patent level with a high mortality rate and an elevated Th2 response [15].
Our study suggests that there is a normal functioning immune response in infected mice in which both arms of Th1 and Th2 provide flexibility and balance to control the parasites during the course of infection. However, in the virulent infection, the predominant Th1 response is not adequate to save all mice from death. However, recent studies showed that in addition to Th1 and Th2 subsets, Th17 and T regulatory subsets and cytokines such as IL-10 and TGF β, are also involved in the regulation of the immune response to malaria [51] and other infections such as toxoplasmosis [52] and schistosomisis [53]. These recent findings show other different fields which merit to be investigated in malaria parasites infections in order to understand true interactions which form the final outcome of the immune responses in either single or mixed malaria infections.
ACKNOWLEDGEMENTS
We should thank the Ministry of Health of I. R. Iran for its financial supports and all who helped us at the Division of the Infection and Immunity at the University of Glasgow, UK.