Differential Expression of Hox and Notch Genes in Larval and Adult Stages of Echinococcus granulosus
Article information
Abstract
This investigation aimed to evaluate the differential expression of HoxB7 and notch genes in different developmental stages of Echinococcus granulosus sensu stricto. The expression of HoxB7 gene was observed at all developmental stages. Nevertheless, significant fold differences in the expression level was documented in the juvenile worm with 3 or more proglottids, the germinal layer from infected sheep, and the adult worm from an experimentally infected dog. The notch gene was expressed at all developmental stages of E. granulosus; however, the fold difference was significantly increased at the microcysts in monophasic culture medium and the germinal layer of infected sheep in comparison with other stages. The findings demonstrated that the 2 aforementioned genes evaluated in the present study were differentially expressed at different developmental stages of the parasite and may contribute to some important biological processes of E. granulosus.
INTRODUCTION
Cystic echinococcosis (CE) is a parasitic infection caused by the larval stage of the dog tapeworm Echinococcus granulosus. It is found in the small intestines of carnivores, and the eggs are passed via fecal matter. CE has been identified as an important economic and public health concern in developing countries [1]. Humans and domestic livestock can be intermediate hosts, in which the worms form hydatid cysts in various organs [2]. Differentiation into either a strobilated cestode in the dog intestine or a hydatid cyst in visceral or non-intestinal sites of intermediate hosts is one of the unique properties of E. granulosus. This phenomenon indicates unexpected biological features of the worm and presents a special field of study to investigate the basic issues in Platyhelminthes differentiation [3].
Studies on the in vitro cultivation of Echinococcus species show that the parasite is a suitable model for differentiation. Depending on the culture conditions, protoscoleces can either develop in cystic or adult directions. Several investigations have reported that controlling the capacity of protoscoleces into adult worms or hydatid cysts is associated with the host’s environmental conditions such as the contact of evaginated protoscoleces with a suitable protein-containing substrate [3–5]. In addition to the influence of environmental conditions such as pH, oxygen tensions, and bile salt concentration, the molecular basis of bidirectional development needs to be investigated. Whole-genome sequencing, proteomic and transcriptional investigations have identified genes and proteins that are differentially expressed between the different life stages and cyst components of E. granulosus [6–8]. However, the identities of some genes responsible for the main molecular events that lead to structural developments of E. granulosus and its evolution between various developmental stages are unknown.
The Homeobox B7 (HoxB7) gene family has emerged as important master regulators of development. They are present in the genomes of all animals, have been highly conserved throughout evolution, and are directly involved in the morphological diversification [9,10]. Notch signalling pathway is found in organisms as diverse as worms and humans. The notch gene plays a critical role in tissue development, regulation of cell proliferation, and differentiation and cell fate in all metazoans [11,12]. However, there are no molecular developmental studies on HoxB7 and notch genes in E. granulosus. The purpose of the present study was to evaluate the differential expression of these genes in terms of fold differences in the germinal layer of hydatid cyst in sheep as an intermediate host and the microcysts grown in monophasic culture media.
MATERIALS AND METHODS
Collection of protoscoleces
The protoscoleces of hydatid cysts were obtained from the livers of naturally infected sheep slaughtered at Kerman abattoir, southeast of Iran. The hydatid fluid was aspirated aseptically using a 50 ml syringe, and then the germinal layer was removed. The layer was washed 4 times and stored at −80°C until testing. Subsequently, the collected protoscoleces were washed 4 times with sterile PBS. The number of protoscoleces per ml was adjusted as 1×104 protoscoleces in 0.9% NaCl solution with more than 90% viability rate. The viability of the protoscoleces was confirmed by their flame cell motility and impermeability to 0.1% eosin solution under a light microscope. Afterwards, the parasite was genotyped as described elsewhere [4,13–15]. The protoscoleces were digested in 1% (w/v) pepsin prepared in 0.85% (w/v) NaCl (pH 4.0) for 30 min at 37°C to release protoscoleces from the brood capsules. Lastly, pepsin-digested protoscoleces were used for 3 assays; (i) in vitro culture in diphasic media to reach adult form, (ii) in vitro culture with feeder cells for microcyst formation, and (iii) in vivo adult worm formation by experimental infection of a dog.
In vitro cultivation of protoscoleces in diphasic medium
In vitro cultivation of protoscoleces into E. granulosus adult worm in diphasic medium was carried out as described by Smyth et al. [16] with some modifications. The culture medium was S.10E.H, which consisted of 2 phases: (1) liquid phase containing 260 ml CMRL 1066 medium (Thermo Fisher Scientific, San Jose, California, USA), 100 ml heat inactivated fetal calf serum (FCS; Gibco-BRL, Gaithersburg, Maryland, USA), 36 ml 5% yeast extract (Sigma-Aldrich, St. Louis, Missouri, USA) in CMRL 1066, 5.6 ml 30% glucose (Sigma-Aldrich) in distilled water, 1.4 ml 5% dog bile in PBS, 20 mM HEPES (Sigma-Aldrich), 10 mM NaHCO3 (Sigma-Aldrich) supplemented with penicillin (100 IU/ml), streptomycin (100 mg/ml) (Sigma-Aldrich), and (2) bovine serum coagulated at 76°C for 20–30 min as a solid phase [4,5,17]. Different stages in culture medium were isolated based on the morphological classification described by Smyth et al. [16]. The isolated stages were stored in RNAlater (QIAGEN GmbH, Hilden, Germany) at −80°C until RNA extraction.
In vitro cultivation of protoscoleces in monophasic medium
To reach E. granulosus microcysts, protoscoleces were washed 3 times in Hanks Balanced Salt Solution (HBSS) and added to Dulbecco’s minimal essential medium (DMEM) (Thermo Fisher Scientific) containing 2 mM glutamine (Sigma-Aldrich), penicillin (100 IU/ml), and streptomycin (100 mg/ml) supplemented with 10% heat inactivated FCS. For feeding the protoscoleces during subsequent passages, 1×106 Hepa 1–6 (ATCC® CRL-1830™) was added to each 200 ml flask. The flasks containing the protoscoleces were placed in an upright position in an incubator at 37°C with 5% CO2, and the medium was changed every 4 to 8 days [13,18].
Experimental infection in a dog
This study was conducted with approval from the Research Ethical Review Committee of Kerman University of Medical Sciences (permit no. 92/274). To establish the experimental infection in a dog, a healthy male mixed-breed dog (6 months old) was obtained from a supplier in Kerman city, Kerman Province, Iran. The animal was treated with praziquantel (10 mg/kg body weight) prior to the study commencement and kept in an individual cage to adapt to the living condition and diet. The dog was fed commercial dog food and water ad libitum. After anthelmintic treatment and subsequent microscopic stool examination verifying the negative coprological findings, the dog received approximately 10,000 viable protoscoleces of E. granulosus orally with a meal, and all subsequent food (administration once daily) was previously heat inactivated [19,20].
The dog was euthanized by intravenous barbiturate overdose (Thiopental Nesdonal® Biochemie GmbH, Vienna, Austria) 45 days after the infection and necropsied to isolate E. granulosus adult worms. The small intestine of the dog was opened longitudinally, and the sections were immersed in warm saline. Detached worms and intestinal contents were passed through sieves. The collected worms were washed 3 times with sterile PBS and kept in RNAlater at −80°C until testing [20].
Analysis of mRNA expression by real-time qPCR
Total RNAs were isolated from the following parasites at different developmental stages in culture media: [A] evaginated protoscoleces (up to 1 day post cultivation), [B] juvenile worms with excretory canals and bladder formation (5–7 days), [C] juvenile worms with first proglottid formation (18–22 days), [D] juvenile worms with second proglottid formation (26–32 days), [E] juvenile worms with testes and genital pore appearance (34–36 days), [F] juvenile worms with 3 or more proglottid formation prior to embryonated egg production in diphasic culture medium, and [G] microcysts in monophasic culture medium. In addition, total RNAs were extracted from the following ex vivo stages: [H] invaginated protoscoleces, [I] germinal layer of hydatid cyst from infected sheep, and [J] adult worms isolated from the experimentally infected dog. Total RNAs from various stages were isolated using the RNeasy mini kit (QIAGEN GmbH) and the contaminating genomic DNA was removed from the RNA samples with DNaseI in a column digestion according to the manufacturer’s protocol. The quantity and quality of the RNA samples were assessed using the NanoDrop ND-1000 spectrophotometer (NanoDrop Technologies, Wilmington, Delaware, USA). Samples with concentrations >100 ng/μl, A260/A280 ratio between 1.8–2.0 and A260/A230 ratio of 1.7–2.0 were retained.
Total RNA (1 μg) was used to synthesize the first cDNA strand for each sample using the Omniscript® Reverse Transcriptase kit (QIAGEN GmbH) with random primers (Sigma-Aldrich) and a final volume of 20 μl. The final cDNA products were diluted 50 times with nuclease free water before qPCR.
Gene sequences obtained from the EMBL and GenBank databases were used to design specific primers for HoxB7 (EUB63018) and Notch (CDJ25139): HoxB7 (EgHoxB7_F GCGAACGCATCTGCGGG and EgHoxB7_R TTCTTCACCTTCATTGGAGG) and Notch (Egnotch_F: TCTGTCTACGGTGACGGTG and Egnotch_R: ACAGCACGGAGAAGGTCTG). The amplicon size was 237 and 215 bp, respectively.
Relative quantitation using SYBR green PCR Master Mix (QIAGEN GmbH) using 7.5 μl of diluted cDNA (1:50) as template per 15 μl reaction was performed using the Rotor-Gene Q (QIAGEN GmbH) as per the manufacturer’s instructions. The reaction conditions were initial activation at 94°C for 15 min, 45 cycles of denaturation at 94°C for 10 sec, annealing at 58°C for 15 sec, and extension at 72°C for 35 sec for each primer pair. The point of quantification was adjusted according to the melting curves of each primer pair. A dissociation step from 50°C to 94°C with ramping increments of 0.1°C/sec was added to assess the amplification specificity for each product through melting curve analyses. To determine the PCR amplification efficiency for each gene, standard cDNA dilutions were prepared using 5 two-fold serial dilutions. PCR results were normalized to the levels of TATA-Box binding protein (TBP), elongation factor 1 alpha (EF-1a), and cyclophilin (CYP-1) as reference genes [21]. The expression of all the selected developmental genes was evaluated using ΔΔCT method. There were 4 technical replicates per biological stage for each gene. For each run, a reverse transcription negative control (without reverse transcriptase) for each synthesized cDNA and a non-template negative control were included to confirm the absence of DNA contamination.
Statistical analysis
Data analysis was conducted by SPSS statistical package, version 17.0 (SPSS Inc., Chicago, Illinois, USA). One-way ANOVA test was used to assess the differences between the relative quantities of each gene. In addition, P<0.05 was considered statistically significant.
RESULTS
Genetic characterization
The results of partial amplification of cox1 segment showed a product size of 366 bp. Nucleotide sequences were aligned with the reference sequences of each genotype within E. granulosus retrieved from GenBank. The findings of sequence analysis of the collected protoscoleces demonstrated the presence of E. granulosus sensu stricto (G1 genotype).
In vitro cultttivation and in vivo development of adult worm
The different stages of E. granulosus in diphasic culture medium were observed as follows: intact protoscoleces (Fig. 1A), evaginated protoscoleces (Fig. 1B), excretory canal and bladder formation (Fig. 1C), first proglottid formation (Fig. 1D), second proglottid formation and testes and genital pore appearance (Fig. 1E), and 3 or more proglottids formation (Fig. 1F). Parasite microcysts developed in monophasic culture medium after 45 days, which is depicted in Fig. 1G. The mature adult worms of E. granulosus with 3 proglottids were isolated from the small intestine of experimentally infected dogs 45 days post infection (data not shown).
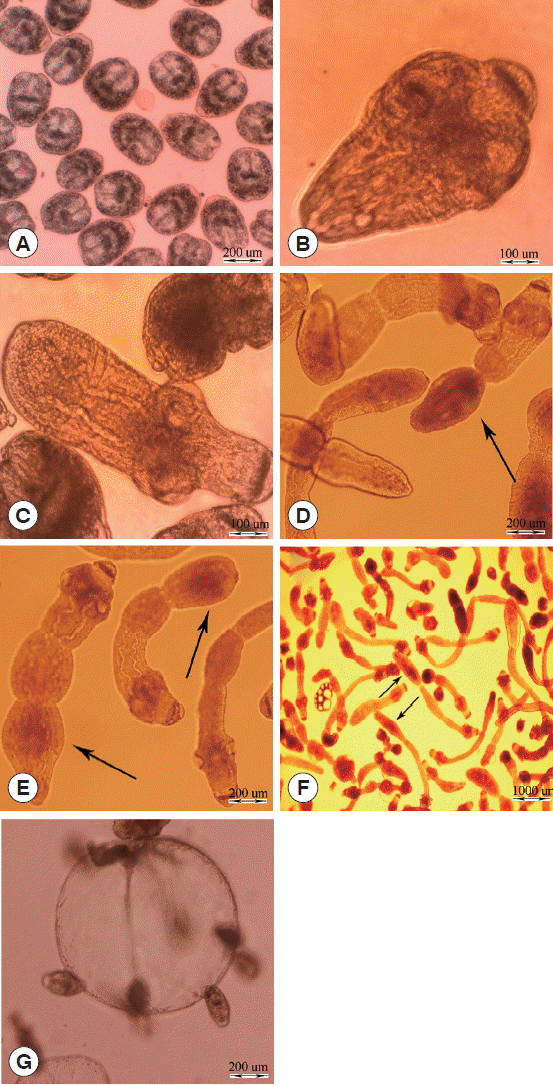
In vitro developmental stages of Echinococcus granulosus in Biphasic (A–F) and monophasic (G) culture media. (A) Intact protoscolex. (B) Evaginated protoscolex. (C) Excretory canals and bladder formation. (D) First proglottid formation. (E) Second proglottid formation. (F) Third or more proglottid formation. (G) Microcyst cultivated in vitro.
Notch gene
The results showed that notch gene was expressed at all developmental stages of E. granulosus (Fig. 2). Nevertheless, the level of expression was significantly upregulated at vesicle (31.3-fold) and germinal layer (86.4-fold) stages in comparison with the other stages (P<0.001).
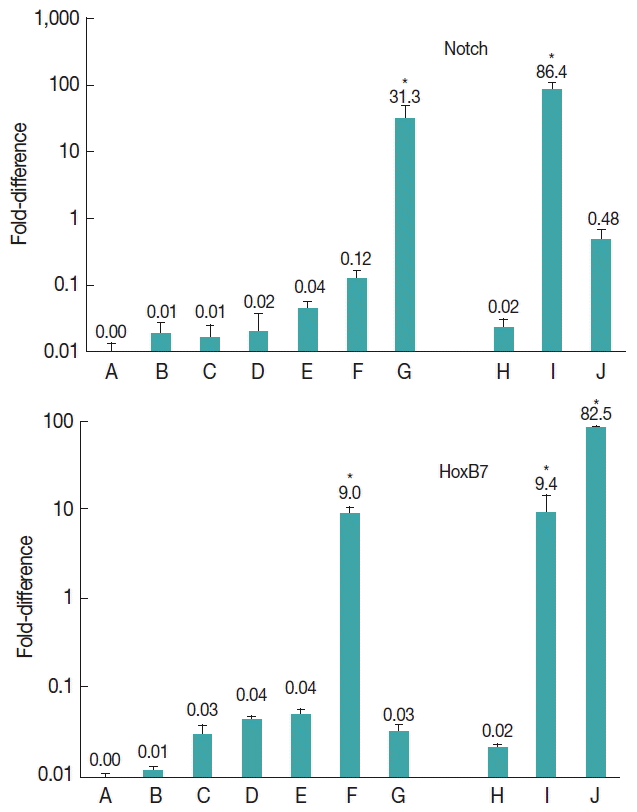
Expression of Notch and HoxB7 genes in developmental stages of E. granulosus in vitro and in vivo. (A) Evaginated protoscoleces. (B) Excretory-canal-and-bladder-formation. (C) First-proglottid-formation. (D) Second proglottid-formation. (E) Testes-and-genital-pore-appearance. (F) Third-or-more-proglottid. (G) Microcysts in monophasic media. (H) Intact protoscoleces. (I) Germinal layer of hydatid cyst. (J) Adult worms isolated from a dog. *statistically significant (P<0.05).
HoxB7 gene
The expression of HoxB7 gene was observed at all developmental stages of E. granulosus (Fig. 2). The level of expression was significantly upregulated 9.0-fold in 3 or more proglottids formation (P<0.05), 9.4-fold in germinal layer (P<0.05) and 82.5-fold in adult worm stages (P<0.001).
DISCUSSION
In vitro cultivation of E. granulosus has been undertaken for last few decades in order to study the developmental biology, differentiation, and host-parasite relationships [3,5]. In this study, we obtained different developmental stages of E. granulosus in biphasic and monophasic culture media (Fig. 1). Moreover, we established the experimental infection in dogs to reach the fertile adult worm. The differential expression of HoxB7 and notch genes were evaluated at different developmental stages (in vivo and in vitro) of the parasite, including 7 in vitro stages of strobilization and microcysts formation as well as 3 ex vivo stages, including intact protoscoleces, germinal layer, and the adult worm in the experimentally infected dog.
The HOX subgroup of the homeobox supergene family consists of several genes that encode transcription factors with the capacity to activate or repress downstream target gene expression. HOX genes also regulate growth and differentiation during embryonic development and maintain adult tissue homeostasis. In addition, the Hox family, which is characterized by its highly conserved DNA binding domain, has been identified in almost all metazoans. This gene family is considered important in the study of developmental biology [9,10]. HoxB7, a member of the HOX family, encodes a protein with a homeobox DNA-binding domain and functions as a sequence-specific transcription factor [22,23]. HoxB7 participates in processes such as cell proliferation and differentiation and anterior/posterior pattern formation [24]. Hox genes are involved in body segment identity in the fruit fly Drosophila melanogaster [25]. Hox genes were originally identified as key regulators of body segment of invertebrates. Moreover, an important role of HoxB7 is its involvement with morphological diversification of various body structures during development [26].
The presence of Hox gene family members has been investigated in a number of parasitic tapeworms (e.g. Taenia asiatica, Hymenolepis microstoma, Mesocestoides corti, and Echinococcus multilocularis) as well as free-living flatworms (e.g. Schmidtea mediterranea) [27–30]. In the present study, all developmental stages of E. granulosus showed some degree of HoxB7 expression. Our findings revealed the overexpression of HoxB7 gene in 3 or more proglottids, the germinal layer and the adult worm, suggesting a probable role of this gene in cell differentiation and proliferation of E. granulosus. The germinal layer of hydatid cysts contains cells that are actively involved in proliferation and differentiation. The upregulation of HoxB7 at this stage may be attributed to this process. A dramatic increase in HoxB7 expression was demonstrated at the adult stage of the parasite, which correlates with the role of the gene in body segmentation. Findings of the present study showed that a high level of HoxB7 expression has been observed in late stages of strobilization; however, further investigations are needed to elucidate other possible elements affecting this process.
The Notch signalling pathway is an evolutionarily conserved cell signalling system present in most multicellular organisms. Notch operates in many cell types and at various stages during development. In addition, it regulates numerous developmental processes [31]. Notch activity modulate multiple aspects of metazoan development and has recently been linked to stem cell fate and maintenance in adult tissues [32]. Previous studies on the nematode Caenorhabditis elegans have demonstrated that Notch signalling plays a critical role in the development and induction of mesoderm and cell fate specification [12]. Furthermore, Liu et al. [32] reported that Notch signalling plays various fundamental roles during multiple stages of organismal development, in lineage-specific differentiation of pluripotent embryonic stem cells and in controlling stem cell numbers and their activity. Deng et al. [33] demonstrated that the Notch pathway controls the mitotic-to-endocycle transition in Drosophila follicle cells. In the present investigation, a 31.3- and 86.4-fold upregulation of notch was observed in microcysts and germinal layer stages, respectively. The findings of the present study showed that notch was significantly upregulated only in the germinal layer and vesicle/microcyst formation, suggesting a probable role of notch gene products in the mitotic cell division and proliferation of E. granulosus.
ACKNOWLEDGMENTS
The authors would like to thank the technical and administrative staff of the Institute of Parasitology, University of Bern, Switzerland and the Department of Parasitology, School of Medicine, Kerman University of Medical Sciences, Kerman, Iran. The authors would like to thank Katherine Nixon, Iran Virayesh Center, for the English revision and editing of the manuscript.
Notes
CONFLICT OF INTEREST
We have no conflict of interest related to this work.